 |
Supercontinent tectonics | |
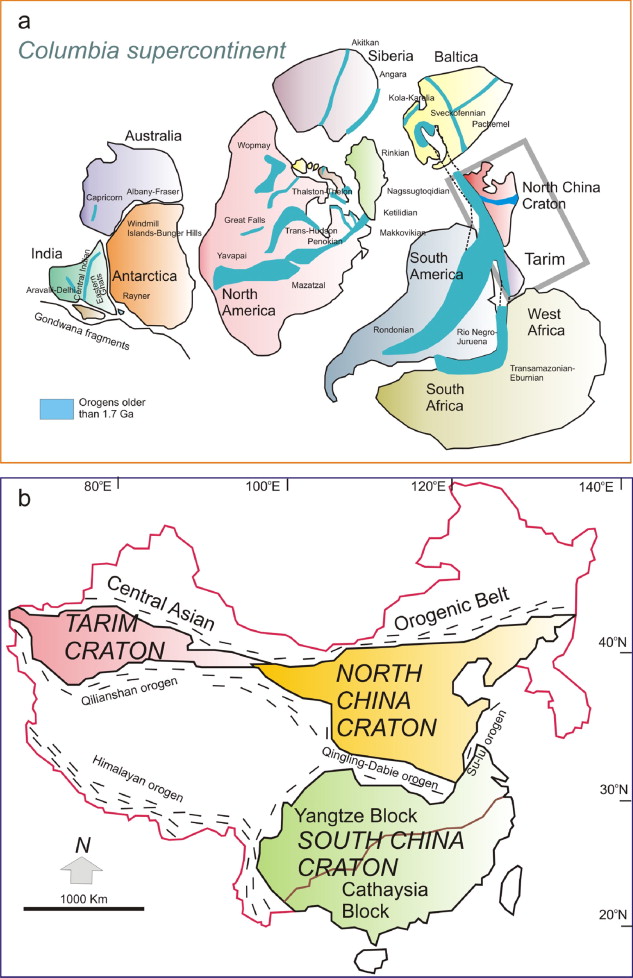
a) North China Craton within the Paleoproterozoic Columbia supercontinent (after Kusky and Santosh, 2009). (b) Tectonic framework of China illustrating the major Precambrian cratons and younger orogens (after Zhao et al., 2005).http://www.sciencedirect.com/science/article/pii/S0301926810000446
|
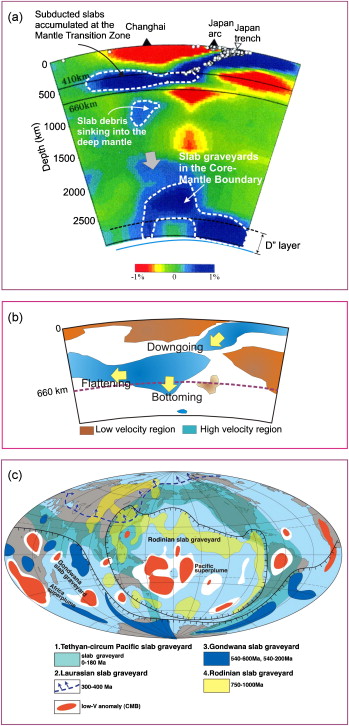
(a) P-wave tomography of the Western Pacific region showing slab graveyards (after Zhao, 2004). The vertical cross-section is shown for a profile passing through northeast China and central Japan. The velocity perturbation scale is shown at the bottom. The flat-lying stagnant slabs in the mantle transition zone extends for over 2000 km. The low velocity anomalies under the Pacific slab in the upper and topmost lower mantle, if representing a compositional anomaly, might indicate subducted felsic material. See text for discussion. (b) Sketch from P-wave tomographic image beneath Izu-Bonin arc showing the anatomy of subducted slab including the downgoing, bottoming and flattening domains (after Fukao et al., 2009). (c) Speculated slab graveyards on the coreиCmantle boundary through geological time as proposed by Maruyama et al. (2007) from P-wave whole mantle tomography by Zhao (2004). The calculated slab graveyard back to 180 Ma are also shown superposed with the present day distribution of continents. The green region represents slab graveyard during the last 180 Ma, the yellow region shows the Rodinia slab graveyard, and the dark blue region shows the Gondwana slab graveyard. The region with arrows shows the slab graveyard of Laurasia. The definitions of the slab graveyards were based on the paleopositions of the supercontinent or megacontinent. The Pacific superplume occurs at the center of the Rodinia slab graveyard. The African superplume is also shown within the Gondwana slab graveyard (after Maruyama et al., 2007)
|

Cartoon speculating the fluid distribution from surface to the core of the Earth (after Santosh et al., 2009b and references therein). Mid oceanic ridge basalt (MORB) subducted at the trench sinks through the mantle transition zone and finally drops down to the coreиCmantle boundary (CMB). The MORB components are then heated up by the outer core leading to partial melting. The dense iron rich melts accumulate on the bottom of the Dбх layer. The remaining restite MORB with dominant andesitic composition rises upward to form superplume. The subducted slab from the trench is hydrous, and is heated up by the surrounding mantle which releases the water in the mantle wedge and enhances the viscosity. The origin of a superplume at the coreиCmantle boundary is discussed in Maruyama et al. (2007). The vertically rising superplume enters into the upper mantle, transforms to horizontal and branches out into several hot spots. These hot spots cause the rifting of the continent, and deliver the mantle fluid (mainly C-O-H-S) to the surface. Surface CO2 has been selectively transported into the mantle in the Hadean to the Archean. After the Neoproterozoic, surface water started to be transported into the mantle transition zones (410иC660 km). For the major part of the Earth''s fluid history, the fluid transport was mostly one wayбкfrom the outer core to the surface. The return flow of water is thought to have been initiated probably after 750 Ma, and has not yet entered into the lower mantle. MORбкMid ocean ridge.
|

Cartoon illustrating the process of material circulation on a whole-earth scale controlled by plate, plume and anti-plate tectonics (after [51] and [88]). The subducted slab material, as inferred from seismic tomography, may be plausibly considered to reach the coreЦmantle boundary. This recycled oceanic lithosphere is viewed as a potential trigger of, and contributor to, the superplume rising from the coreЦmantle interface to the uppermost mantle, penetrating the mantle transition zone and eventually giving rise to hot spots. Radioactive heat generated in СenrichedТ basaltic slab remnants, and the heat given off by post-perovskiteЦperovskite phase transformation may contribute to generating small-scale plumes which ultimately coalesce into a superplume (see Maruyama et al., 2007 for details). The implication of horizontal movement at the base of the mantle has been referred to as Сanti-plate tectonicsТ, in many respects analogous to lithospheric plate tectonic processes operating in near-surface regions. Accordingly, through time, it is conjectured that continents gradually develop at or close to the outer earth surface while concomitant Сanti-continentsТ would be generated at the CMB (Maruyama et al., 2007). Also shown are tectosphere-bearing surface continents and water subduction into the mantle boundary layer (mantle transition zone) where rising hydrous plumes are predicted.
|
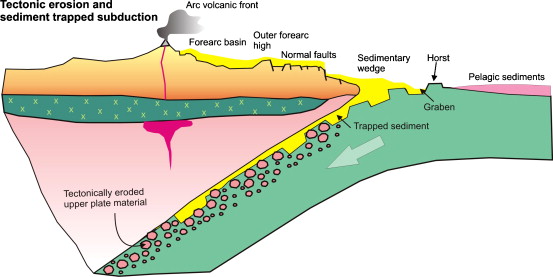
Cartoon illustrating tectonic erosion and sediment trapped subduction
|
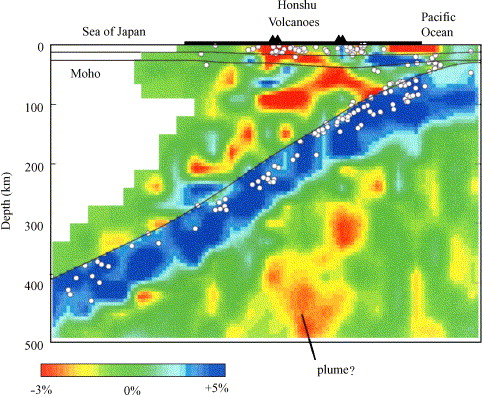
|
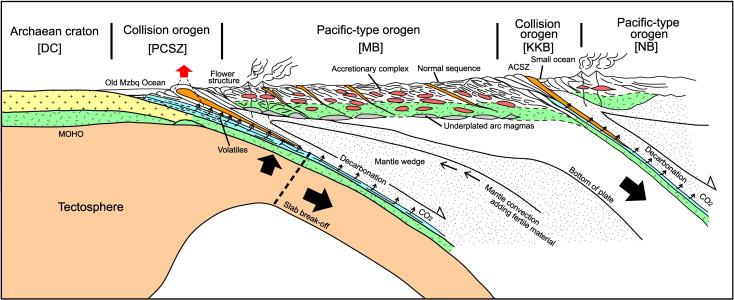
Cartoon illustration showing a composite plate tectonic model for southern India from North (Archean Dharwar Craton) to South (Pacific-type orogen) with two major collisional orogens in between. The PCSZ represents the main suture developed through Mozambique Ocean closure. The cross section also covers ACSZ + KKB/Trivandrum Block up to the Nagercoil Block at the southern tip. The Madurai Block represents a wide magmatic arc in between.Anatomy of a Cambrian suture in Gondwana: Pacific-type orogeny in southern India? -http://www.sciencedirect.com/science/article/pii/S1342937X09000057
|
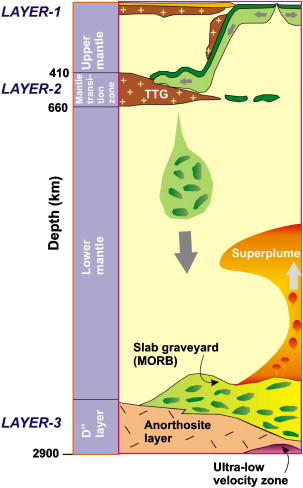
Cartoon speculating the dynamics and compositional layering of solid Earth through time (after Kawai et al., 2009). The anorthositic crust formed after the consolidation of magma ocean in the Hadean was ultimately subducted to the bottom of the mantle with time. Simultaneously, TTG crust began to accumulate at the mantle transition zone, as the buoyancy of the material inhibited its transport further deep into the mantle. The model predicts that through time, TTG crust has accumulated in the mantle transition zone, whereas the subducted mid oceanic ridge basalt together with slab peridotites dropped down to accumulate on the coreЦmantle boundary. Thus a three-layer structure can be speculated on a whole Earth scale with substantially larger СcontinentsТ and СsupercontinentsТ occurring on the mantle transition zone and coreЦmantle boundary as compared to those on the surface of the globe
|
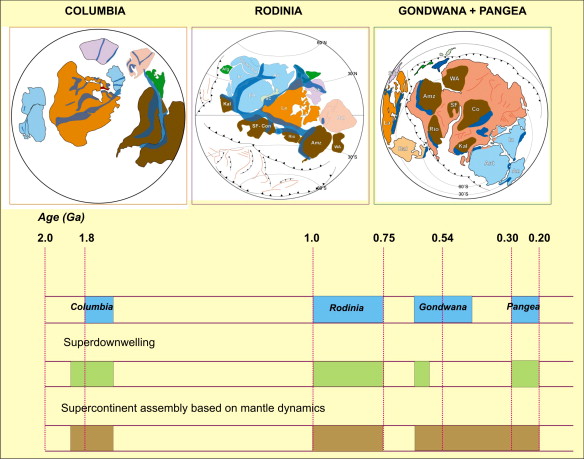
History of major supercontinents speculated from mantle dynamics (after Senshu et al., 2009). The top panel shows supercontinents through time from the Paleoproterozoic Columbia (which is perhaps the first coherent supercontinent) at ca. 1.8Ц1.9 Ga through Rodinia at 1.0 Ga, and a combination of Gondwana (540 Ma) and Pangea (250 Ma). The bottom panel shows the timing of super-downwelling events, a process that is considered as critical for the rapid amalgamation of continents and as evaluated based on the presence of Y-shaped triple junctions from paleogeographic configurations of supercontinents (Santosh et al., 2009a). The super-downwellings would therefore roughly correspond to the timing of assembly of supercontinents. Based on the configuration, super-downwelling and mantle dynamics, the only supercontinent that was assembled within a short period of time was the hypothetical Paleoproterozoic supercontinent Columbia. The three parameters also match well for Rodinia. However, there is a wide time gap between Gondwana and Pangea, and therefore Senshu et al. (2009) clubbed these and proposed that, the timing of a true supercontinent assembly subsequent to the Neoproterozoic Rodinia in terms of mantle dynamics, is at ca. 340 Ma. According to this model, the lifespan of supercontinents becomes longer with time which has been correlated to the decrease in the rate of heat generation by subducted TTG material.
|
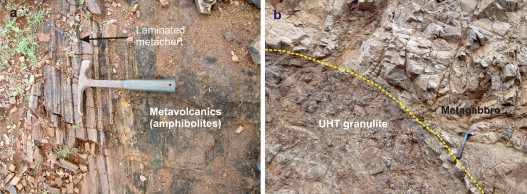
Representative field photographs illustrating evidence for subduction and ocean closure preserved in the deeply eroded collisional sutures of Precambrian supercontinents where diagnostic lithologies considered as markers for sutures are absent. (a) Field photograph showing the association of amphibolites (metavolcanics) and metachert from the Palghat-Cauvery Suture Zone, a trace of the Late Neoproterozoic Gondwana-forming suture. This association and other various evidences from this zone (discussed in Santosh et al., 2009c) provide support for ocean plate subduction during the closure of the Neoproterozoic Mozambique Ocean. (b) Field photograph showing the association of ultra-high temperature (UHT) MgЦAl granulite and metagabbro from the Inner Mongolia Suture Zone in North China Craton ( [86] and [90]), a trace of the Paleoproterozoic Columbia suture. The association of UHT granulite and metagabbro within duplexes suggest extreme metamorphism of MgЦAl sediments within the subduction channel and their extrusion and accretion together with remnants of the oceanic lithosphere and/or fragments of the mantle wedge during the final collisional stage
|
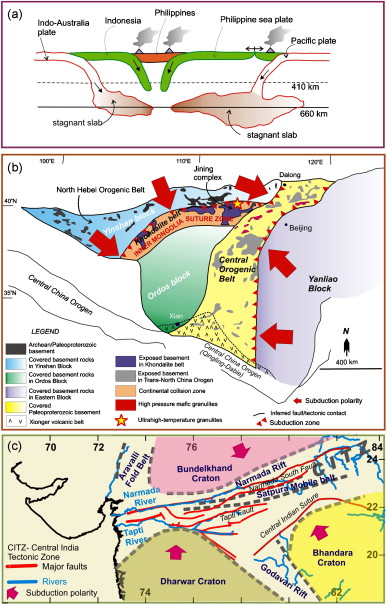
(a) Cartoon illustrating the concept of double-sided subduction discussed in the text (after [51] and [87]). (b) Geological and tectonic framework of the North China Craton showing the assembly of crustal blocks through opposing subduction as interpreted from a recent synthesis of S-wave receiver functions, S-wave velocities and two versions of P-wave tomographic images along various transects in this craton (after Santosh, 2010). The assembly of the major crustal blocks within the North China Craton during the Paleoproterozoic and their incorporation within the Columbia supercontinent are correlated to double-sided subduction history. (c) The Central India Tectonic Zone (CITZ) that sutures the South and North Indian blocks. The major cratons are also shown. A combined geological and geophysical synthesis suggests double-sided subduction as a trigger for the Mesoproterozoic suturing event (after Naganjaneyulu and Santosh, in press).
|
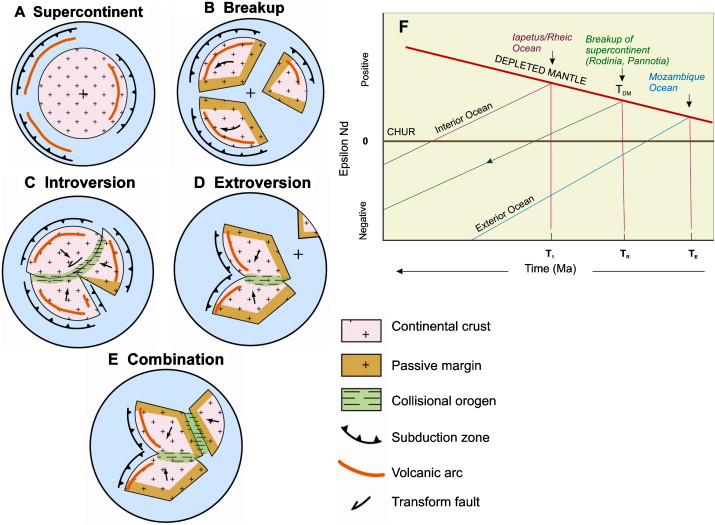
(AЦE) Stages of breakup and assembly of supercontinents through СintroversionТ, СextroversionТ or a combination of both introversion and extroversion as discussed in the text (after Murphy and Nance, 2005). (F) Schematic representation of the Sm/Nd isotopic evolution of oceanic lithosphere from the interior and exterior oceans (from Murphy et al., 2009). The depleted mantle ages for the interior ocean (TI) are younger than the time of supercontinent breakup (TR). Whereas in the case of exterior ocean (TE) the ages are older. Relative to the breakup of Rodinia supercontinent, the Mozambique Ocean is an exterior ocean; relative to the breakup of Pannotia, the Iapetus and Rheic Oceans are interior oceans
|
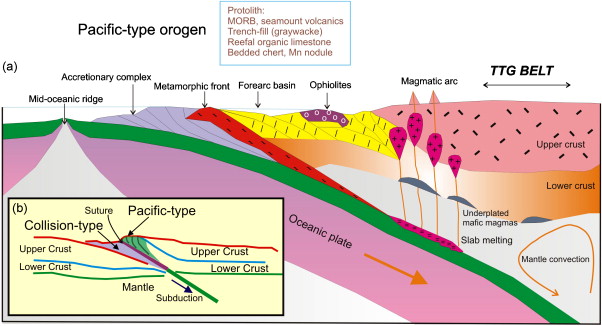
Cartoon sketch illustrating the anatomy of a Pacific-type orogen (modified after Maruyama and Parkinson, 2000). The inset figure shows paired Pacific-type and collision-type orogens that develop on either side of the suture during continental amalgamation as proposed in this study.
|
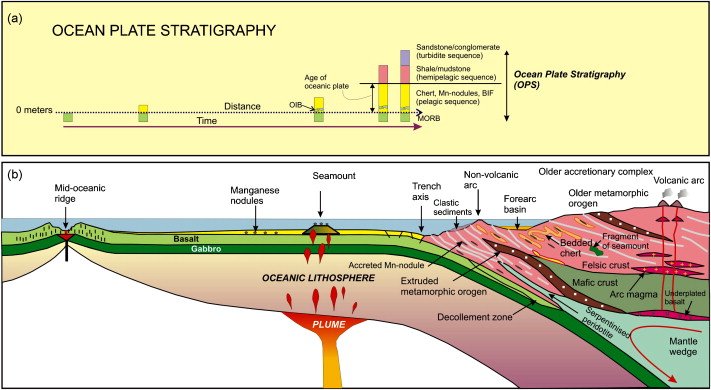
Sketch showing the travelogue of an oceanic plate from its origin at the mid oceanic ridge to its subduction at the trench to illustrate the concept ocean plate stratigraphy (OPS) (with information synthesized from [54], [63] and [53]). The top panel shows the characteristic original stratigraphic succession developed during successive stages interpreted as a function of distance from the ridge and time of travel
|
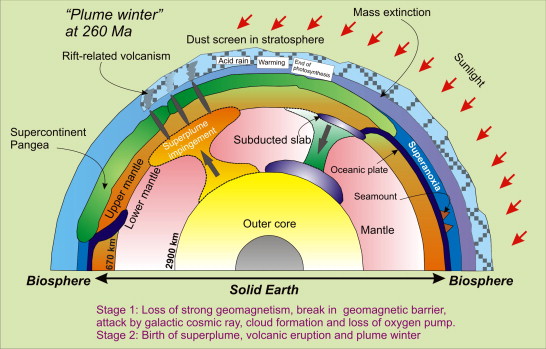
Cartoon illustrating the role of mantle dynamics and supercontinents in life history and surface environment. The figure shows the relationship between superplume, supercontinent breakup and mass extinction (after Isozaki, 2009) during the Late Guadalupian (Middle Permian; ca. 265 Ma) event, an episode termed the Illawarra Reversal. The model envisages two stages: (1) a strong retardation in the geomagnetic field led to a break in the geomagnetic barrier allowing galactic cosmic rays to infiltrate. This resulted in the formation of thick cloud layers which ultimately stopped the oxygen pump. The second stage is marked by the birth of a superplume and its upwelling, followed by voluminous volcanic eruption leading to plume winter
|
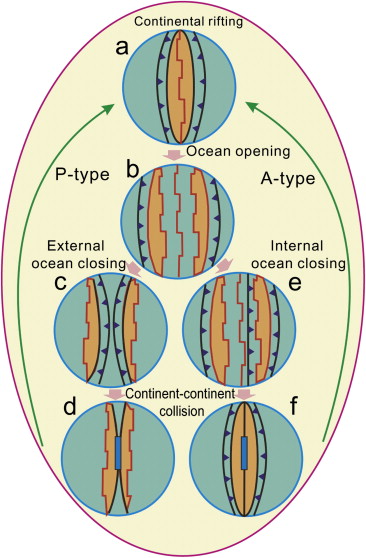
Two types of ocean closure following supercontinent breakup as proposed by Silver and Behn (2008). Orange-shaded regions Ц supercontinents; black lines with dark blue triangles Ц subduction zones; thick red lines Ц internal ocean; blue rectangles in (d) and (f) Ц oceanic crustal materials including ophiolites trapped in the suture zone. http://www.sciencedirect.com/science/article/pii/S167498711000006X
|
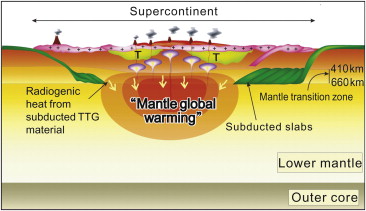
Schematic illustration of the thermal blanket hypothesis of supercontinent breakup (after Senshu et al., 2009).http://www.sciencedirect.com/science/article/pii/S167498711000006X
|
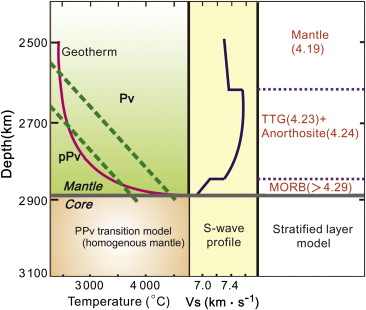
Phase relations for continental crust represented by anorthosite and TTG (tonalite-trondhjemite-granodiotite; after Komabayashi et al., 2009). MORBЦMid ocean ridge basalt; PvЦ perovskite; PpVЦ post-perovskite. The typical mantle geotherm is also shown. See text for discussion. The study demonstrates that all these materials can be subducted down to the core-mantle boundary, leading to the development of a compositional stratification in the DФ layer
|
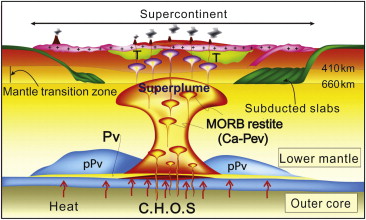
Supercontinent breakup initiated by superplume rising from the core-mantle boundary (after [Maruyama et al., 2007] and [Senshu et al., 2009]). The phase change of subducted Mid Ocean Ridge Basalt (MORB) into post-perovskite releases latent heat generating many small scale hot plumes, which eventually gather to create a superplume
|
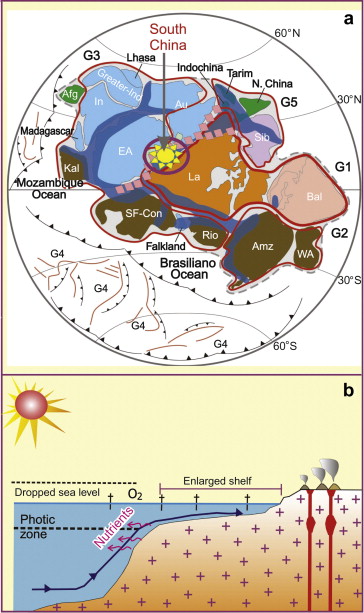
A speculative model for the evolution of modern life forms following supercontinent breakup. The breakup of the Neoproterozoic Rodinia supercontinent (a, after Rino et al., 2008) generated enlarged shallow marine environments (b, after Maruyama and Santosh, 2008), with increased oxygen level and enrichment of nutrients. This isolated environment was conducive for the extensive development of body plans and enhancement in the size and diversity life forms which finally led to the so-called Cambrian explosion. The rift-related basins thus provided the Сlife soupТ for the rapid evolution of modern life. G1 to G5 in the Rodinia reconstruction shown in (a) correspond to the classification into various groups (North American, African, Russian and Asian Grenville Groups) based on Rino et al. (2008) on the basis of zircon age data
|
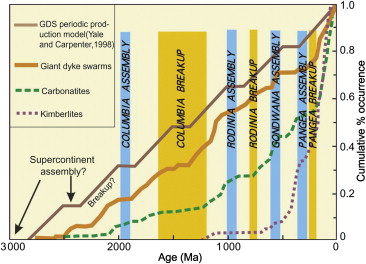
Cumulative percentage of the occurrence of giant dyke swarms, carbonatites and kimberlites and the model periodic production of giant dyke swarms (after Yale and Carpenter, 1998). The approximate timings of assembly and disruption of the major supercontinents through Earth history are also shown.
|
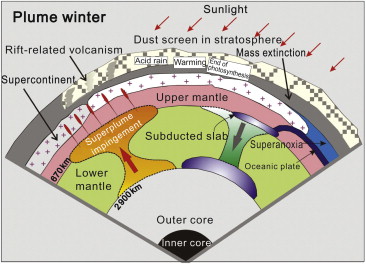
Cartoon illustrating the role of mantle dynamics and supercontinents in life history and surface environment. The figure shows the relationship between superplume, supercontinent breakup and mass extinction (after Isozaki, 2009) during the Late Guadalupian (Middle Permian; ca. 265 Ma) event, an episode termed the Illawarra Reversal. The model envisages two stages: (1) a strong retardation in the geomagnetic field led to a break in the geomagnetic barrier allowing galactic cosmic rays to infiltrate. This resulted in the formation of thick cloud layers which ultimately stopped the Уoxygen pumpФ; the second stage is marked by the birth of a superplume and its upwelling, followed by voluminous volcanic eruption leading to plume winter.
|
From http://www.sciencedirect.com/science/article/pii/S0264370710000700
A synopsis of recent conceptual models on supercontinent tectonics in relation to mantle dynamics, life evolution and surface environment
A synthesis of some of the recent conceptual models suggests that mantle dynamics exerted a significant control on the assembly and breakup of supercontinents through the history of the Earth. During the amalgamation of continental fragments, the subducted oceanic lithosphere of intervening oceans either moves down to the deep mantle or gets horizontally flattened as stagnant slabs in the mantle transition zone. Blobs of these stagnant slabs sink down into the deep mantle and accumulate as slab graveyards at the coreиCmantle boundary. The recycled oceanic lithosphere at the coreиCmantle boundary is thought to contribute potential fuel for generating superplumes which rise from the coreиCmantle interface to the uppermost mantle, penetrating the mantle transition zone and eventually giving rise to hot spots. Multiple subduction zones promote the rapid amalgamation of continental fragments into supercontinents and also act as major zones of material flux into the deep mantle transporting substantial volume of trench sediments and arc crust through sediment subduction and tectonic erosion. Due to buoyancy, the subducted TTG (tonalite-trondhjemite-granite) material is stacked in the mid mantle region and may not sink down to deeper levels. Thus, continents and supercontinents can be speculated to occur as three layers: on the surface of the globe, at the mid mantle region and on the coreиCmantle boundary, with material transfer on a whole earth scale controlled by plate, plume and боanti-plateбп tectonics. Whereas mantle tomography opens windows into the deep Earth, the imbricated remnants of боocean plate stratigraphyбп preserved in accretionary orogens constitute useful geological tools to study subduction-accretion-collision history, particularly in relation to the assembly of older supercontinents on the surface of the globe. The dynamics of supercontinents also impact the origin and extinction of life as well as surface environmental changes. Large scale flow of material and energy through mantle downwelling and upwelling associated with supercontinent assembly and breakup is thought to affect the Earth''s dynamo which would lead to catastrophic environmental changes, sometimes even triggering mass extinction. When a rising plume impinges the base of a supercontinent, the resultant continental rifting, formation of large igneous provinces and volcanic emissions might lead to the initiation of a plume winter, the aftermath of which would be mass extinction and long-term oceanic anoxia. Supercontinent tectonics in relation to mantle dynamics thus provides a key to evaluate the history of evolution and destruction of the continental crust, to understand the history of life, and to trace the major surface environmental changes of our planet.
Keywords:Earth history; Supercontinents; Subduction; Slab graveyards; Tectonic erosion; Superplume; Mantle dynamics; Life evolution; Surface environment
from http://www.sciencedirect.com/science/article/pii/S167498711000006X
The formation and disruption of supercontinents have significantly impacted mantle dynamics, solid earth processes, surface environments and the biogeochemical cycle. In the early history of the Earth, the collision of parallel intra-oceanic arcs was an important process in building embryonic continents. Superdownwelling along Y-shaped triple junctions might have been one of the important processes that aided in the rapid assembly of continental fragments into closely packed supercontinents. Various models have been proposed for the fragmentation of supercontinents including thermal blanket and superplume hypotheses. The reassembly of supercontinents after breakup and the ocean closure occurs through УintroversionФ, УextroversionФ or a combination of both, and is characterized by either Pacific-type or Atlantic-type ocean closure. The breakup of supercontinents and development of hydrothermal system in rifts with granitic basement create anomalous chemical environments enriched in nutrients, which serve as the primary building blocks of the skeleton and bone of early modern life forms. A typical example is the rifting of the Rodinia supercontinent, which opened up an NЦS oriented sea way along which nutrient enriched upwelling brought about a habitable geochemical environment. The assembly of supercontinents also had significant impact on life evolution. The role played by the Cambrian Gondwana assembly has been emphasized in many models, including the formation of СTransgondwana MountainsТ that might have provided an effective source of rich nutrients to the equatorial waters, thus aiding the rapid increase in biodiversity. The planet has witnessed several mass extinction events during its history, mostly connected with major climatic fluctuations including global cooling and warming events, major glaciations, fluctuations in sea level, global anoxia, volcanic eruptions, asteroid impacts and gamma radiation. Some recent models speculate a relationship between superplumes, supercontinent breakup and mass extinction. Upwelling plumes cause continental rifting and formation of large igneous provinces. Subsequent volcanic emissions and resultant plume-induced УwinterФ have catastrophic effect on the atmosphere that lead to mass extinctions and long term oceanic anoxia. The assembly and dispersal of continents appear to have influenced the biogeochemical cycle, but whether the individual stages of organic evolution and extinction on the planet are closely linked to Solid Earth processes remains to be investigated
Keywords: supercontinents; Mantle dynamics; Superplume; Life evolution; Extinction; Cambrian explosion
Supercontinent tectonics and biogeochemical cycle: A matter of Сlife and deathТ
The early Earth was dominated by island arcs in an oceanic realm, but after the formation of the proto-continents through arcЦarc collision and accretion, continental crust was assembled into large land masses. These were again fragmented and re-assembled within newer configurations throughout the latter half of Earth history. The assembly and disruption of supercontinents has had significant impact on the mantle dynamics, solid earth processes, surface environments and the biogeochemical cycle ( [Worsley et al., 1986], [Worsley and Nance, 1989], [Condie, 2001], [Condie et al., 2001] and [Santosh, 2010], among others). Among surface environmental changes is the well established relationship of supercontinents and sea level changes. When continents are packed together, sea level is generally low, as against a high sea level when they are in a dispersed state (Parsons and Sclater, 1977). An increased sea level leads to flooding of the continents, whereas low sea level exposes the continental shelves. Global climatic patterns are also markedly influenced by the formation and fragmentation of supercontinents. When continents are assembled together, an icehouse climate predominates and when they are dispersed, a greenhouse climate takes over. Life evolution is less extensive when continents are welded together, whereas isolated marine environments during continental breakup accelerate extensive diversification (Maruyama and Santosh, 2008).
When continents assemble into large land masses, the weathering processes in the increased surface area consume more CO2 from the atmosphere, which is eventually transferred into the oceans through erosion. Increased weathering and erosion leads to the release of more nutrients which promote enhanced biological productivity. The enhanced CO2 sequestration also leads to cooler climates with more intense ocean circulation, nutrient upwelling, marine productivity and phosphate deposition. The drastic drop in CO2 concentration, together with the increasing albedo caused by the high land/ocean ratio would ultimately result in widespread glaciation. On the other hand, supercontinent breakup leads to the formation of rift basins, and the restricted circulation promotes anoxic conditions in the deeper parts of the basins. Actively eroding escarpments along new rift margins contribute sediments to rift basins, and marine transgressions increase the rate of burial of organic and carbonate carbon on stable continental shelves.
An increase in length of the ocean ridge system during supercontinent breakup would also promote mantle degassing and release of CO2 into the atmosphere (Condie, 2001). The rising CO2 levels and elevated sea level generate warmer climates. Thus, the EarthТs climatic evolution has been tectonically punctuated by alternating intervals of УgreenhouseФ drowning and УicehouseФ emergence. Such icehouse intervals may also correspond to episodes of biotic innovation, and phosphate deposition. Worsley et al. (1986) summarized the recurring and non-recurring events during the evolutionary history of the Earth where continental differentiation, enzyme efficiency, atmospheric oxygen levels, and solar luminosity are considered to represent increasing secular trends. Worsley (ibid.) divided a supercontinent cycle into four phases: fragmentation, maximum continent dispersal, continental assembly, and supercontinental stasis. These recur at ca. 500 Ma interval and correlate with tectonism, cratonic sediment preservation, atmospheric and hydrospheric evolution, and the distribution of marine platform stable isotopes. Non-recurring and irreversible events such as development of photosynthesis, formation of banded iron formations, and deposition of detrital uraninite represent periods of rapid geochemical adjustment to biospheric evolution.
This paper provides a synoptic overview of some of the current speculative models on the tectonics of the assembly and disruption of supercontinents and their impact on EarthТs biosphere.
2. Conceptual models on the assembly and disruption of supercontinents
2.1. Supercontinent assembly
In the early history of the Earth, the collision of parallel intra-oceanic arcs was an important process in building embryonic continents. Recent studies from Archean terranes in different parts of the world have offered important clues for the process of amalgamation of composite arcs ( [Komiya et al., 2002] and [Santosh et al., 2009], and references therein). A modern analogue for the Archean process is the western Pacific domain where 60%Ц70% of island arcs are concentrated. In a recent study, Maruyama et al. (2009) described the characteristics and processes of subduction zone magma factories in relation to the age of the subducting plate and the mode of subduction in the western Pacific region.
Based on the topology of Y-shaped triple junctions in major supercontinental assemblies, Santosh et al. (2009) recognized two distinct categories of subduction zones on the globe: the Circum-Pacific subduction zone and the Tethyan subduction zone. In the scenario where the subduction is double sided, the triangular regions with Y-shaped topology selectively refrigerate the underlying mantle and turn down the temperature in these domains as compared to the surrounding regions. The Y-shaped domains also accelerate the refrigeration through larger amounts of subduction and thus promote stronger downwelling as compared to other regions of the mantle. Once this process is initiated, a runaway growth of cold downwelling occurs which eventually develop into a large zone of super-downwelling. Santosh et al. (2009) proposed that such super-downwelling could be one of the fundamental processes that drags the dispersed continental fragments through mantle convection and close-packs them within a tight supercontinent assembly
The effects of supercontinents on the dynamics and structure of the mantle have been addressed in several investigations (e.g., [Gurnis, 1988], [Lowman and Jarvis, 1993], [Yoshida et al., 1999], [Coltice et al., 2007], [Phillips and Bunge, 2007] and [Zhang et al., 2009]). These studies identify that a mantle convection planform with short-wavelength structures and a large number of downwellings may not lead to the assembly of supercontinents. This is because continental blocks would be trapped by different downwellings, thus inhibiting collision. If the continental blocks floating on the Earth are smaller than the wavelength of mantle flow and their number is larger, it would take a longer time for them to form a supercontinent ( [Phillips and Bunge, 2007] and [Zhang et al., 2009]). Thus, long-wavelength convection is preferred for effective close packing of continental fragments into a supercontinent assembly. Both Rodinia and Pangea were largely surrounded by subduction zones suggesting downwellings and upwellings, and leading to kinematic models of supercontinent cycles which require mantle convection of very long-wavelengths.
Whereas Уsupercontinent cycleФ describes the periodic assembly and disruption of land masses, the УWilson cycleФ refers to the opening and closing of large oceans. Although both are considered as complimentary, the processes associated with the assembly, dispersal and reorganization of supercontinents are more complex. Hoffman (1991) proposed the concept of Уinside-outФ process as a mechanism of assembling crustal fragments after the breakup of an earlier supercontinent. If the supercontinent was rifted on one side to bear a passive margin such as in the case of the Atlantic Ocean, then the opposite side becomes a consuming boundary along the continents, such as in the case of the Pacific margin. With time, the Pacific Ocean would shrink and finally close by the passive collision of two continents to generate a large continental mass. In the case of Atlantic, initially both continental margins are passive, but later turn to active margins; the ocean shrinks in size and may totally disappear. On the other hand, the Indian Ocean has two different types of margins simultaneously in operation, active and passive, transporting the northern continental margin of Gondwana by the Atlantic-type process and amalgamating the rifted continents to the southern margin of Asia by the Pacific-type process. Therefore, the Indian Ocean-type process illustrates simultaneous continental breakup and continental amalgamation exemplifying the Уinside-inФ mechanism (Murphy and Nance, 2005). Similar simultaneous rifting and accretion on different margins were also proposed in the case of the Paleoproterozoic supercontinent Columbia by [Rogers and Santosh, 2002] and [Rogers and Santosh, 2004]. The Tethyan process started at least by the Permian to Triassic time and operated simultaneously with the Pacific process to the east. Subsequently, double-sided subduction started to define the frontier of the future supercontinent (Maruyama et al., 2007). The Tethyan region is an exam
next
Supercontinent tectonics and biogeochemical cycle: A matter of Сlife and deathТ |