 |
West American Offshore | |
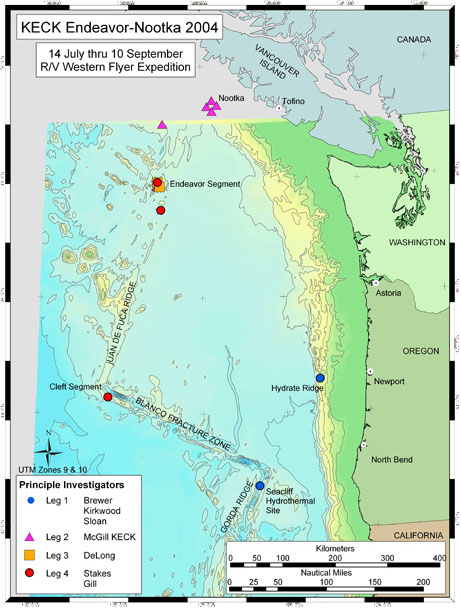
The ultimate principal objective is to test the hypothesis that plate tectonically modulated microbial productivity at the bottom of the ocean may be a significant fraction of the photosynthetically generated productivity near the top of the ocean. At present there is no data on the seafloor sources. Our near-term approach is to develop and deploy sensors that allow simultaneous detection of deformation, fluid/chemical fluxes, and microbial output associated with three types of plate boundaries adjacent to one another. The northern boundaries of the Juan de Fuca plate are the focus of the effort for three reasons: 1) they are spatially condensed, well-defined examples of a ridge, a transform fault, and a subduction complex, 2) they are close to major ports, and, 3) the entire experiment will be central to the Phase I installation by Canada of NEPTUNE.
|
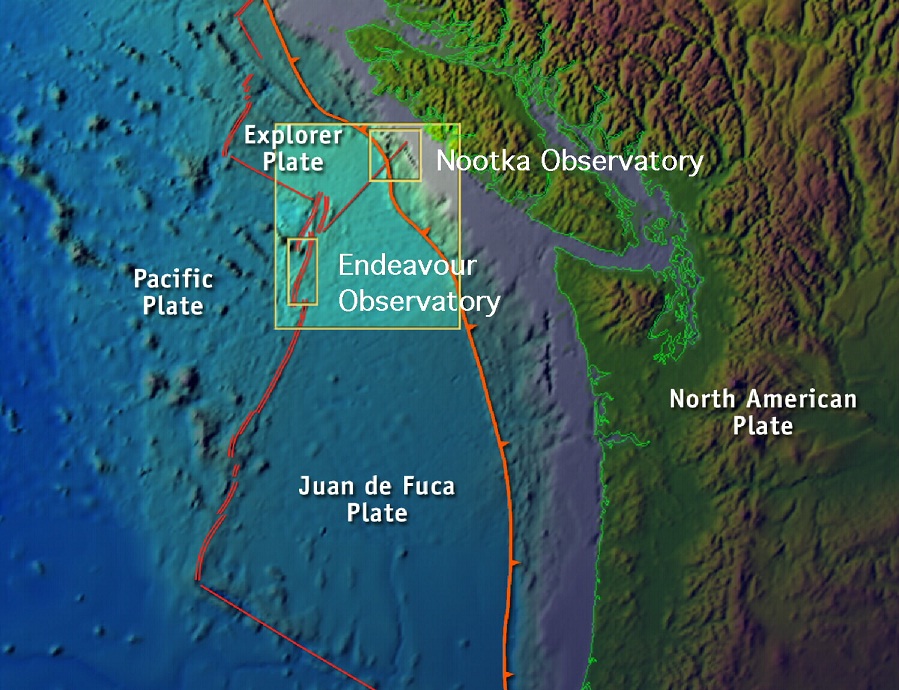
MBARI -http://www.mbari.org/expeditions/WestCoast/index.htm
|
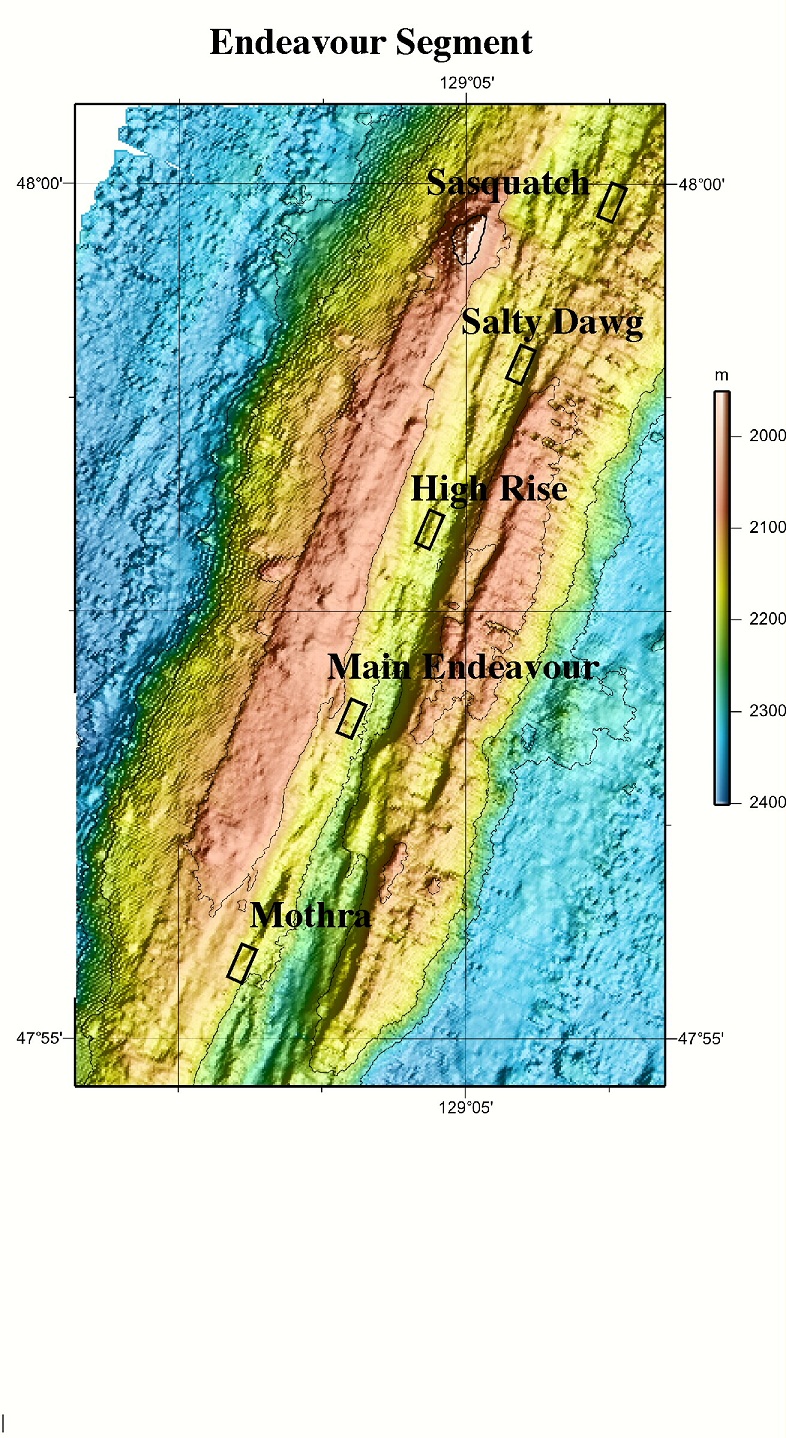
The third leg is funded by the Keck Foundation and will be guided by Dr. John Delaney (University of Washington). The goal of the Keck project is to contemporaneously measure earthquakes, fluid flow and microbial activity across the northern Juan de Fuca plate to assess their interrelationships. The two sites that will be instrumented are the Endeavour Segment of the Juan de Fuca Ridge and the intersection of the Nootka Fracture Zone and the Cascadia Subduction zone. This is the first field effort of this project and the locations of the seismometer array will be determined during the expedition. Boreholes will be made in the basaltic basement at the Endeavour Ridge to provide well-coupled deployment sites for short-period seismometers. Broadband sensors require a meter of thick sediment for burial and we will also search for deposits 2-3 km from the Endeavour spreading axis. The instruments will be deployed in 2003
|
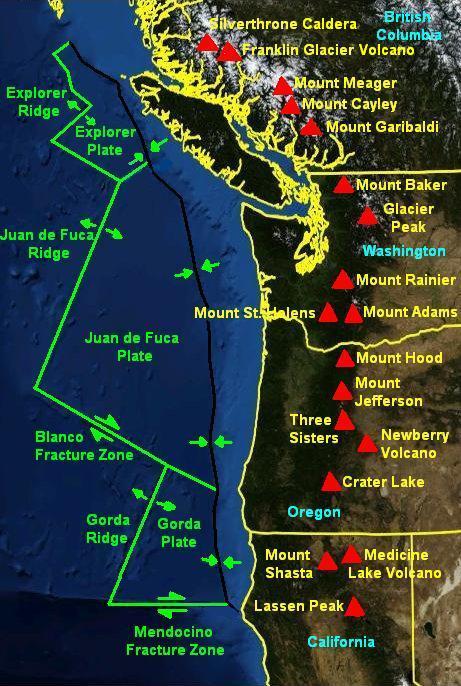
|
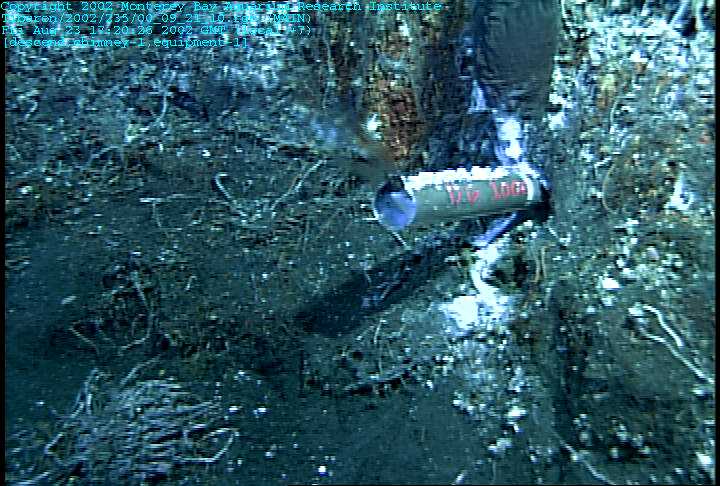
the Mothra hydrothermal site on the southern Endeavour rift valley.Insert protruding from the borehole on Giraffe. The marker makes the site much easier to relocate. Unfortunately little of the insert inside the hole was left after being cooked in the hydrothermal fluids for two days.
|
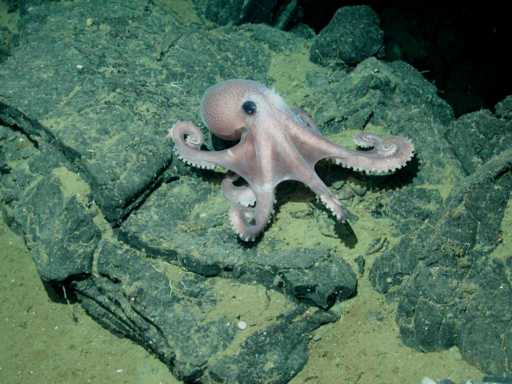
the most common volcanic morphology within the axial valley. These sheet flows are transitional between highly drained lobate pillows and true massive flows. We found similar glassy transitional flows in all of the deeper spots on the axial floor, sometimes separated by fault blocks of more pillowed flows. The protective octopus was less common, but we did see two during this one dive.
|
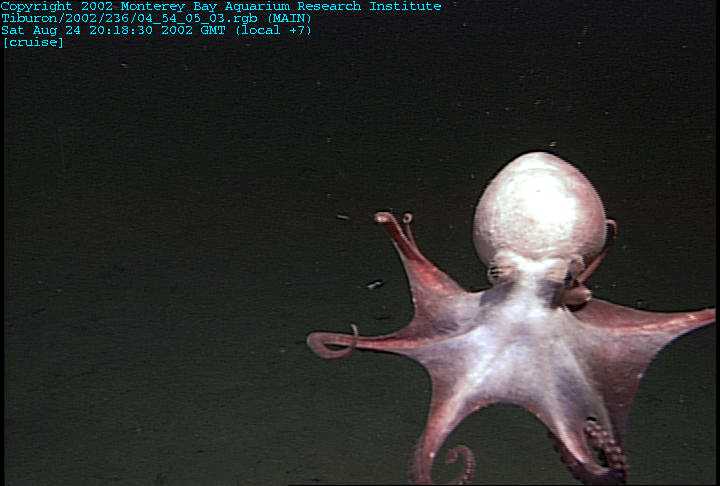
|
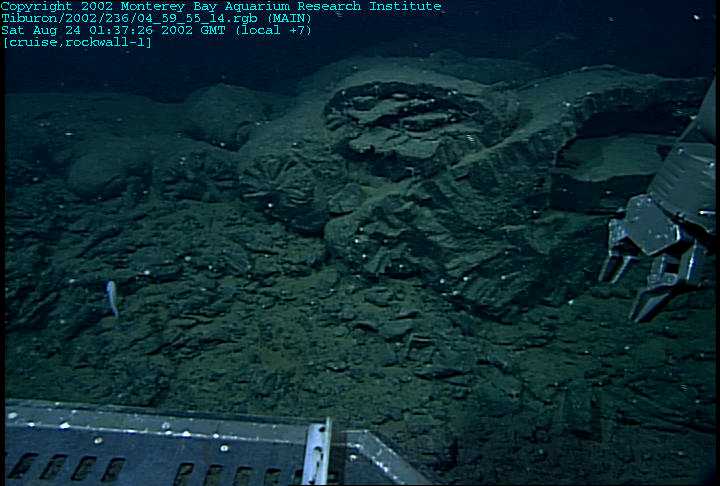
the top of a collapse pit or pressure ridge with fractured pahoehoe flows overrun by lobate pillowed flows
|
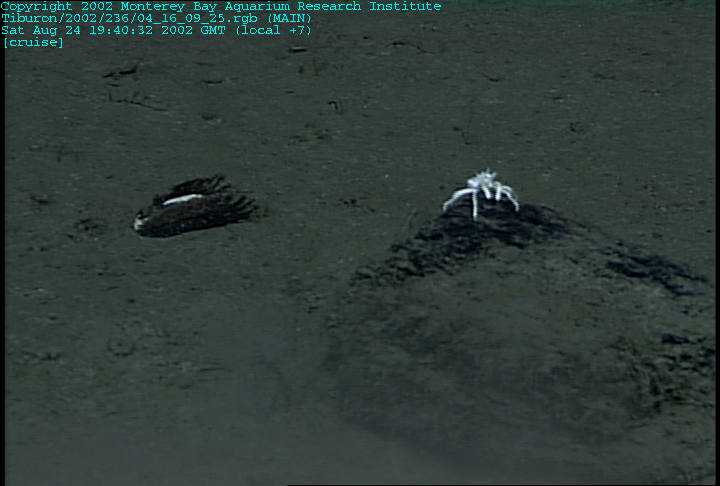
The presence of numerous empty clam shells along with galatheid crabs initially made us hopeful of an easy success
|
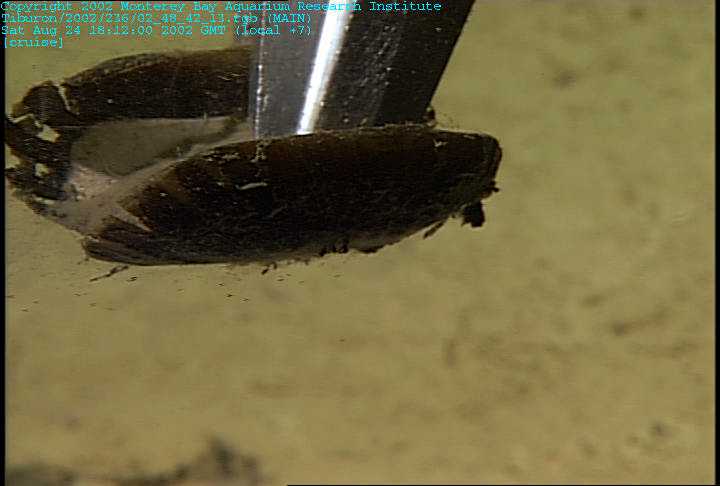
Unfortunately, the only clam shells that we were able to find and collect were empty ones. We found no active seeps and no clams in a living position. There was also no evidence of cold seep carbonates or microbial mat.
|
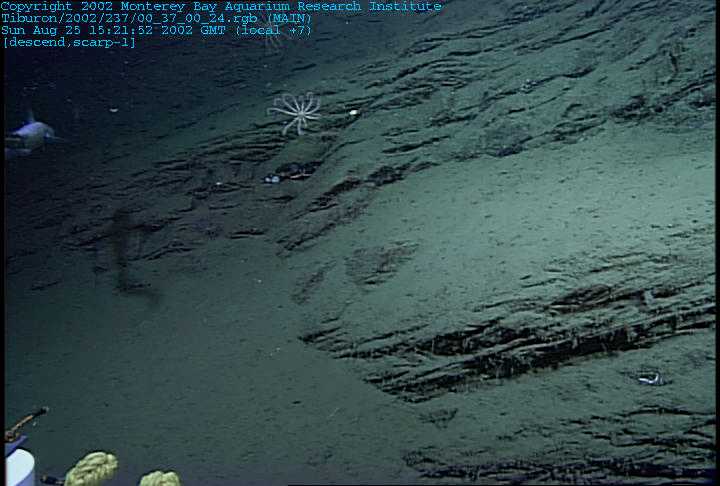
Exposures of tilted and somewhat fractured turbiditic sediments were the norm for the north wall of the Nootka plunge pools
|
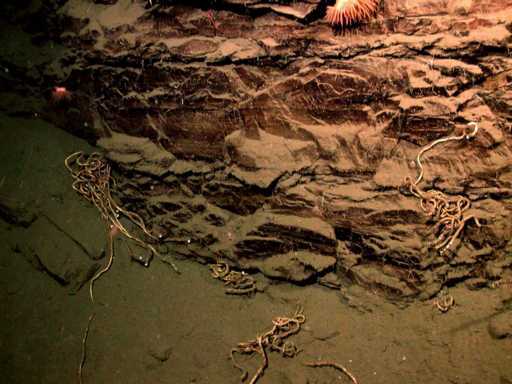
The seepage of sulfide-rich fluids through the fracture network in the sedimentary rocks is evidenced by the clusters of chemosynthetic tubeworms
|
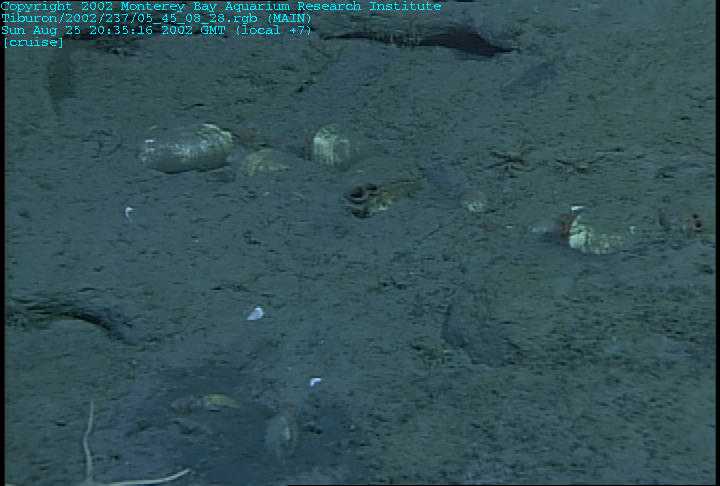
Cold seep clams found near the top of the sedimentary section exposed along the Nootka Fracture Zone plunge pools
|
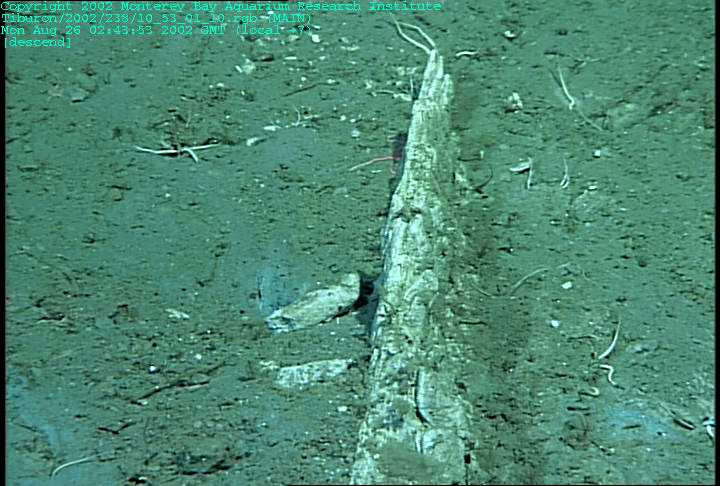
authigenic carbonate infilling the fracture network. The carbonate precipitates from pore fluids that are saturated in bicarbonate, some of which is derived from oxidized methane.
|
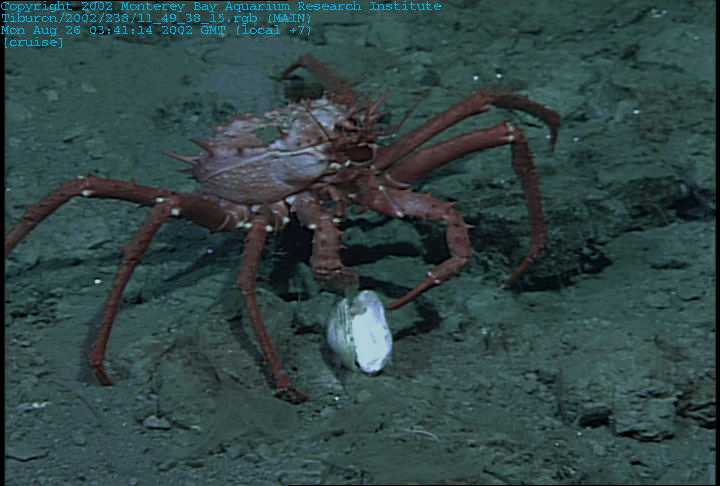
a crab examining a clamshell from the seep.
|
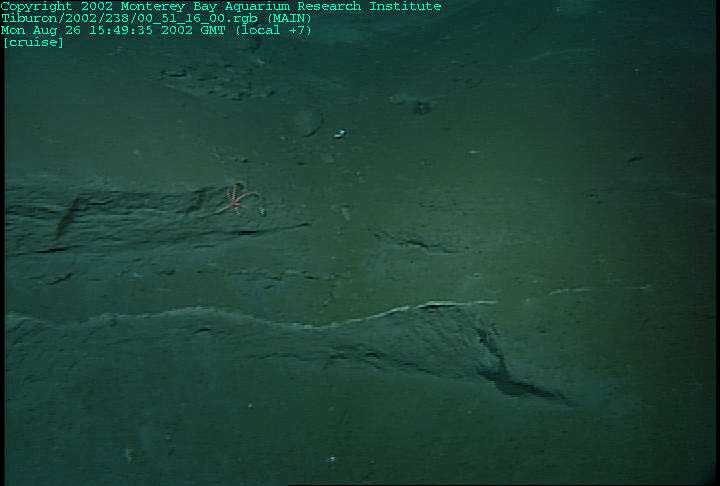
Sediments
|
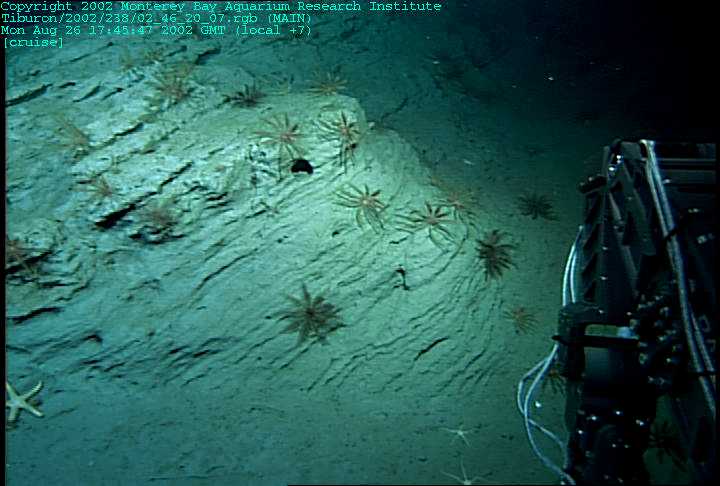
|
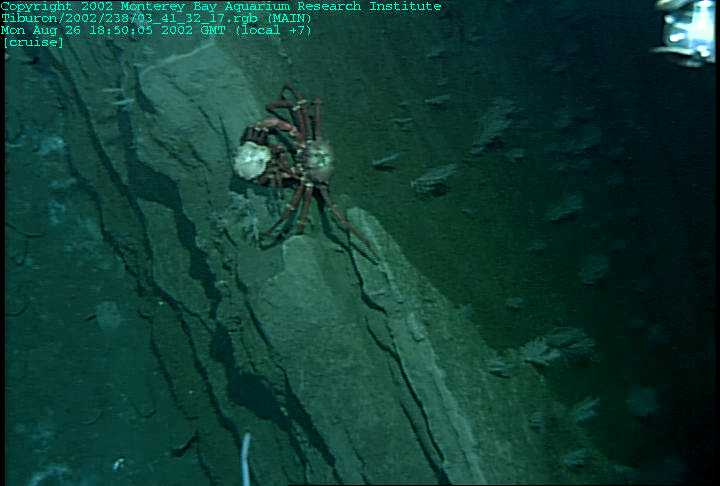
|
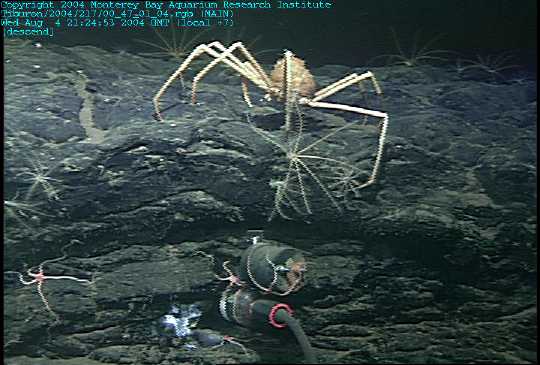
|
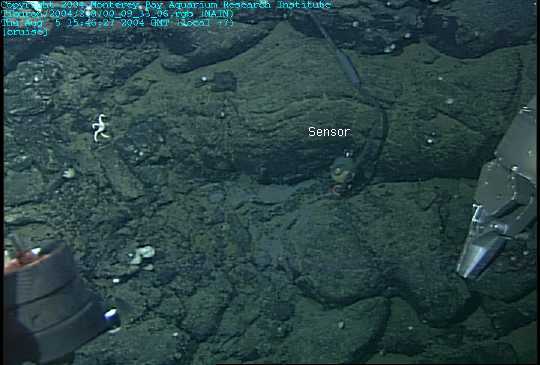
of a small volcanic collapse pit that marks the spreading axis just west of the Sasquatch Hydrothermal Field.Accurate timing is essential to seismology. The reasons for this are easily understood if we consider the nominal speed of seismic waves near the Earth''s surface, which are typically 6.5 to 8 km/s. Using 8 km/s, if the timing of seismic data is accurate to only 1 s, then from a single station the distance to an earthquake can only be measured to within 8 km (i.e., 8 km/s times 1 s). This is a very large and unacceptable error. If timing is accurate to 0.1s, the uncertainty in measuring a distance is about 800 m, or nearly a kilometer. Although this is an improvement, it is still somewhat large as many geologic features can be found within any given kilometer. A typical accuracy of 0.01 s (or 10 milliseconds or ms) yields distance estimates accurate to 80 m, which is clearly an improvement and for many (but not all) applications, acceptable to the average seismologist. As a first cut, it seems pretty obvious that we would like to have a ''time base'' that is accurate at any given time to within 10 ms.
|
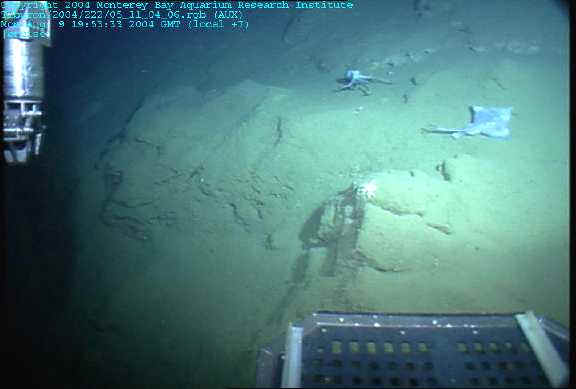
The intersection of the Nootka fault and the Cascadia subduction zone. . A cold seep is the type of vent associated with fluid flow in low temperature environments such as transform zones and subduction complexes. The characteristic seep animals are chemosynthetic tubeworms and clams dependent upon hydrogen sulfide (H2S), similar to communities at hot vents. What I think is very peculiar is that for cold seeps, hydrogen sulfide is not the primary gas coming from the subsurface, as it is in high temperature mid-ocean ridges. Rather, the cold seeps are venting methane (CH3), a gas produced by subsurface microbial breakdown of carbon-rich organic material that has been buried in marine sediments. But none of the animals in cold seeps can directly use the methane for food! Rather, near surface microbial processing oxidizes the methane to dissolved bicarbonate ion combined with the reduction of seawater sulfate to H2S. Microbes called Archea are thought to do the first part of the processing and bacteria accomplish the sulfate reduction. The combination of this microbial processing is to provide H2S to nourish the chemosynthetic biological communities in cold seep environments. A second byproduct is chemical precipitation of calcium carbonate from the sedimentary pore fluids that get saturated with the bicarbonate. So, in environments such as Nootka, chemosynthetic clams and tubeworms along with carbonate precipitates are fingerprints of the up-flow of fluids
|
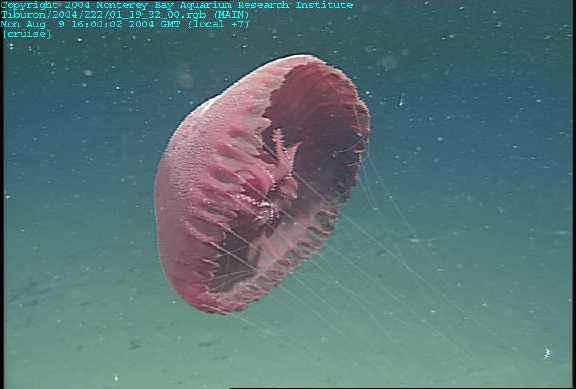
the intersection of the Nootka fault and the Cascadia subduction zone.The edge of the subduction zone is defined by a series of major faults that parallel the coastline. This dive is searching for cold seep communities and carbonates where one of the most western of these faults crosses the Nootka Transform Zone. Much of the fault zone includes dramatically fractured horizons composed of sediments such as mud and sand compressed into rock. Layers of such sedimentary rocks are uplifted and broken into ridges that parallel the subduction zone, where the downgoing plate is first compressed We notice an unusually large number of animals such as octopus, skates, tunicates, crabs, snails, and medusa etc. The abundance of non-seep animals around the seep communities is very reminiscent of my dives on the Mendocino Transform Zone (see 2002 and 2003 dives). Since then I have wondered whether the seeps actually support a broader range of benthic life than just the scattered tubeworms and clams. Finally we find the clam fields, nestled in a muddy pond behind the faulted ridge . An expedition earlier in the summer left the flag to mark the site. We have found the needle in the haystack!
|
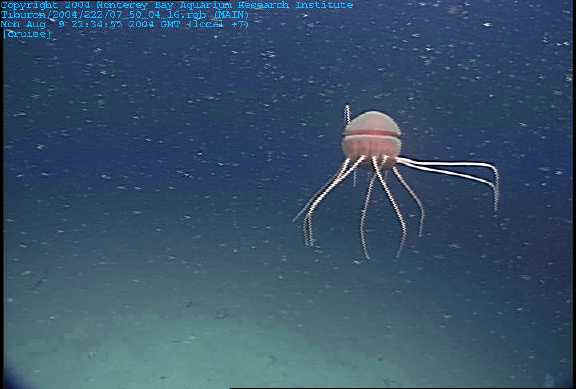
|
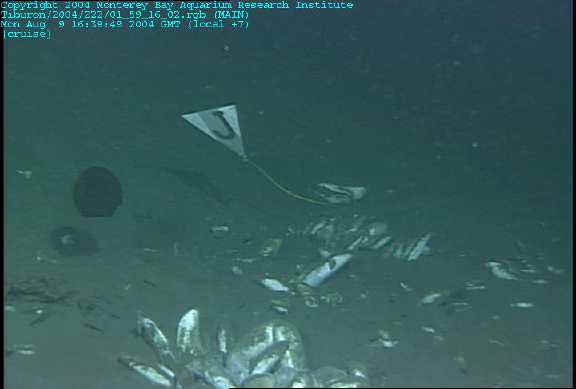
|
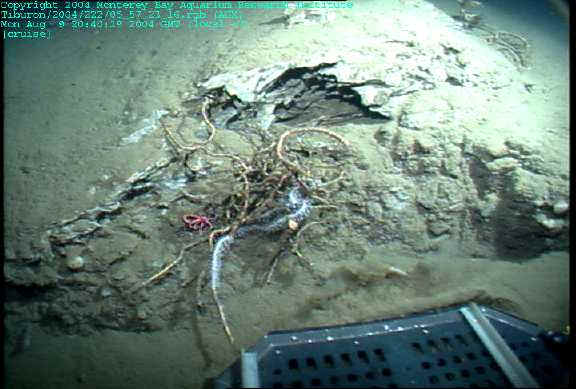
As the ROV moves northward to follow the westward facing wall of the fault, we are amazed to find expanses of tubeworms clinging to the fault face. These look for all in the world like twisted patches of tumbleweeds attached to their plates of carbonate layers. Obviously there are methane-rich pore fluids oozing from the cracks within this escarpment. Our seismometers are in the perfect configuration to monitor the relationship between movement on this series of faults and changes in the size and biological diversity of these communities
|
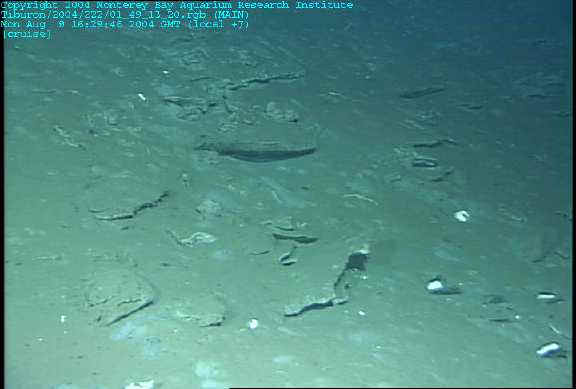
The biologists will study the communities themselves to understand their relationship to others in the area. The geologists and seismologists, in contrast, use the biology as a way to map the distribution and intensity of the underlying fluid flow. After the vehicle has started its trip back to the surface the scientists discuss why the animals were so sparsely and discontinuously distributed. Why is it that there were no chemosynthetic communities right at the intersection of the transform and the subduction zone. Why was one site only clams and the other only tubeworms? There are still so many specific questions to answer before we can address the interrelationships of the larger processes.
|
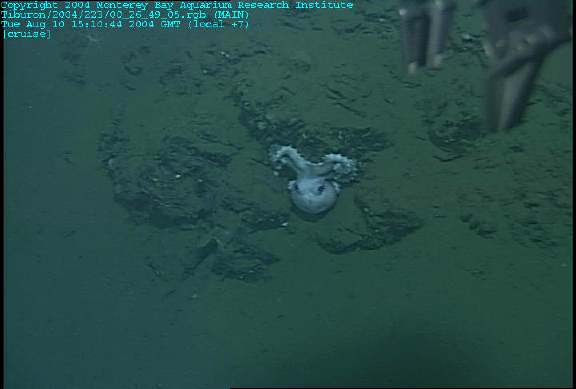
John P. Tully found an interesting mound just in front on the accretionary slices of ocean crust that I described yesterday. On the seismic sections this mound appears to be related to a vertical movement of fluids or fluidized rocks through the ocean crust. Such phenomenon have been related to mud volcanoes or other methane degassing structures. We were given the task of searching for evidence of the origin of this conical structure and determining whether there was any sign of active venting
|
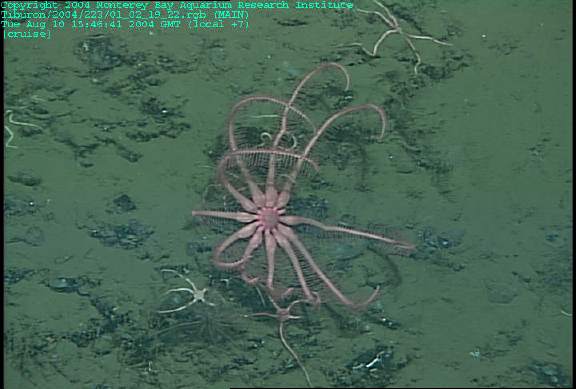
the most likely rock outcrops are to be found on the steepest slopes or the eroded gullies. The flatter base of the structure is all very normal looking lumpy sediment. Even the steepest walls are over 50% sediment-covered. The outcrops that are visible are also heavily populated by all of the normal benthic fauna that prefer to attach to hard bottom. Both sides of the mound had such steeply dipping beds exposed. It was frustrating for me (as a geologist/geochemist) to not be able to take the time to grab a sample to examine on deck. It all looked like terrigenous sediments, but every once in awhile you could peek under a slab and see some provocative broken material of different origin. We patiently looked everywhere for evidence of active fluid flow, but none could be found. A null result may not be the most exciting but it is still important information for the overall program. So I got to call the end of the dive before the very last minute and we cheered, recovered the vehicle, and headed for port.
|
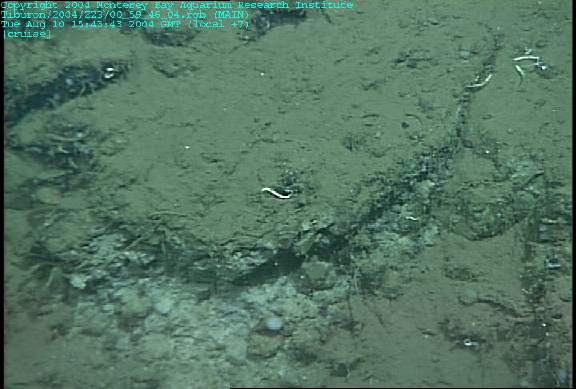
|
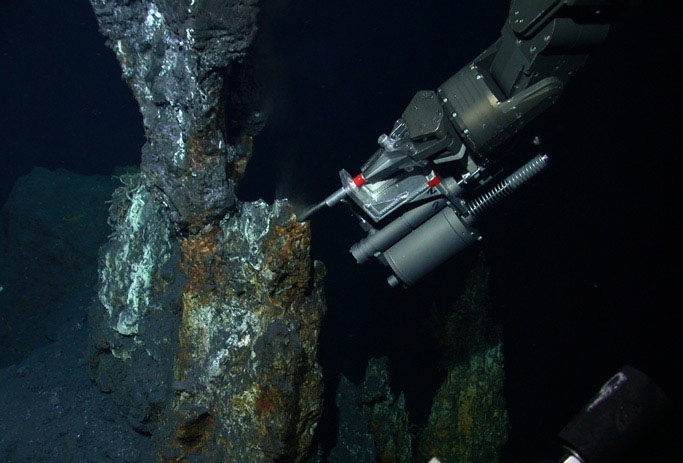
the Endeavour Segment of the Juan de Fuca Ridge, It''s about 200 miles off the coast of Washington and Vancouver Island and is part of a linear volcanic chain, which at the Endeavour is ~2200 m below sea level.The first three dives were located at the Mothra hydrothermal field, the southernmost of the five known fields at Endeavour. Our priorities included the recovery of a microbial incubator that was in the walls of a black smoker chimney, the collection of hydrothermal fluid and rock for microbial studies , samples for vent chemistry and biology, and geological mapping.
|
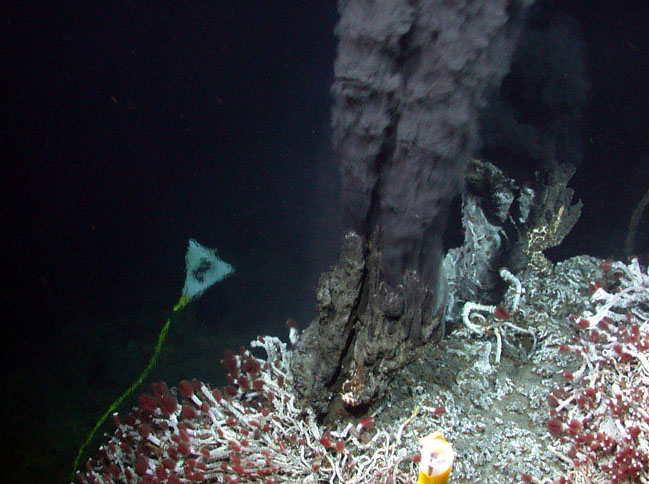
Smocker. Mothra is a great place to study this because there has been a lot of tectonic activity in comparison with some of the other Endeavour vent fields, such as the Main Endeavour Field
|
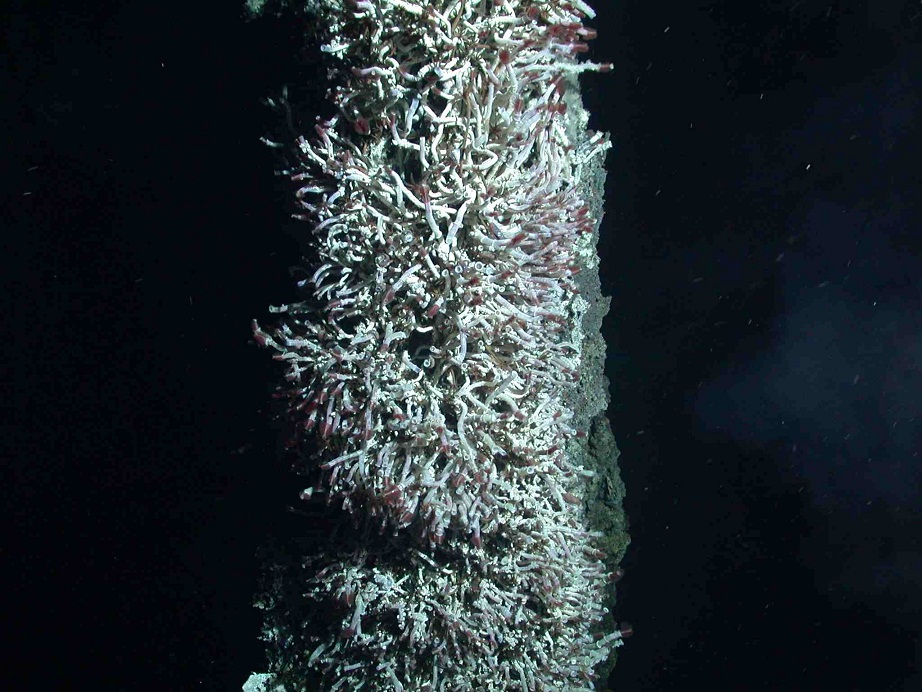
Smoke Mirrors, a huge hydrothermal vent chimney complex in the Main Endeavour field. Its called smoke an mirrors because of all the smokey-looking particles in the water, and because of the mirror-like pools of hot water that collect underneath the flanges (which are pieces of chimney that stick out of the tower) Next, we went to Dudley. Both sites have beautiful, big structures, shapes that couldnt exist on land, and all standing out vividly against the dark, blue water. Some pieces of chimney were broken and collected for scientific observations. Breaking them off sent a cloud of shimmering specks swirling up through the water. We also collected 3 water samples from hot smokers (thats what they call the underwater geysers when they are really focused and really hot), and then set off to explore, climbing up and over and around shelves and up chimneys. The chimney material is very porous in appearance, and is primarily a matte black, with large areas of a shimmery green, which is a copper, iron sulfide, and some white crystallized areas which are calcium sulfate, or anhydride.
|
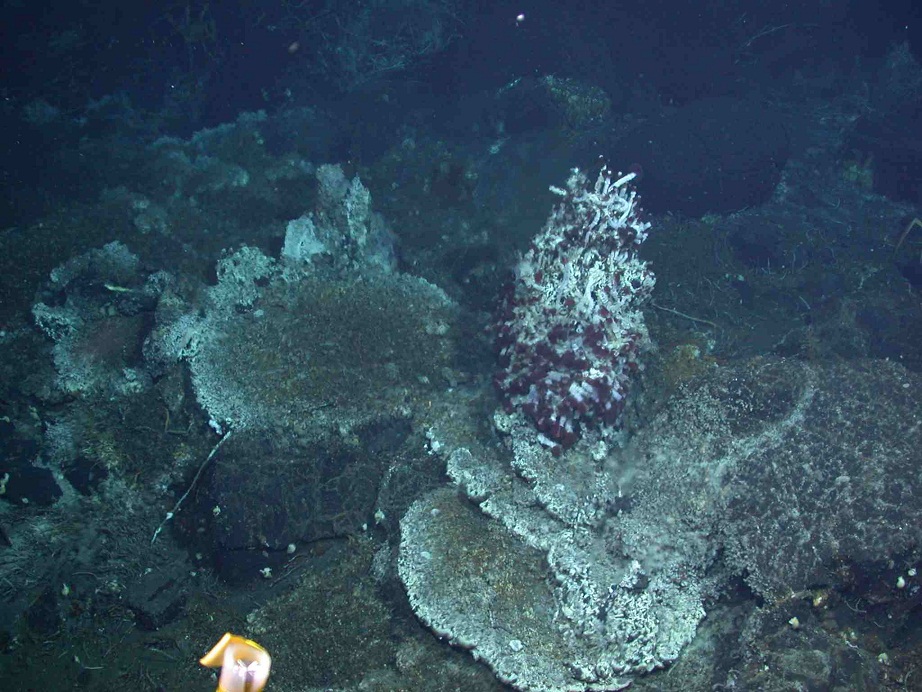
Easter Island, a reef built from hydrothermal venting. Tubeworms and other animals flourish here.
|
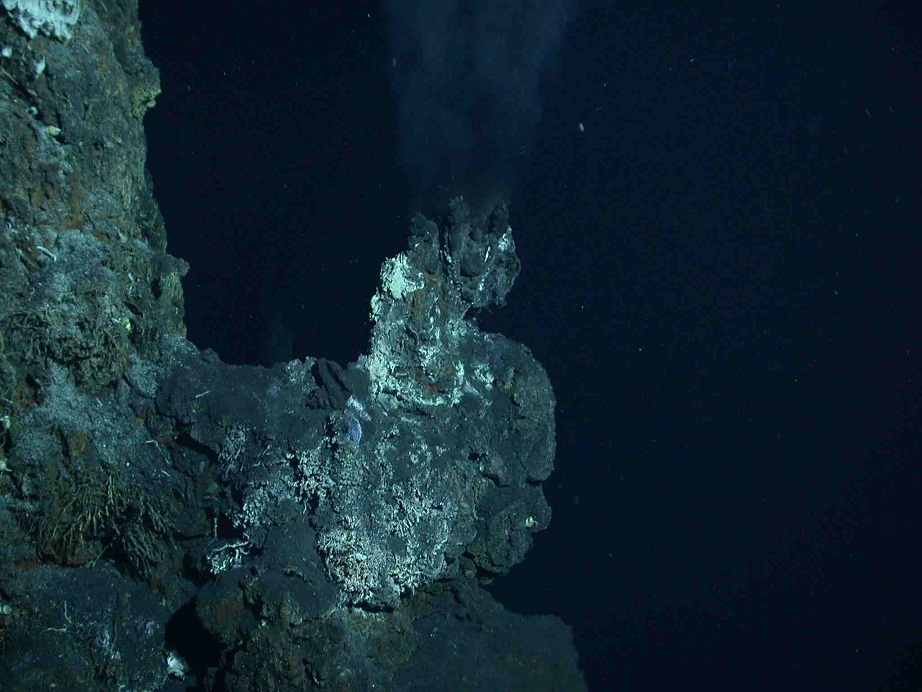
Black smoker on the Faulty Towers complex
|
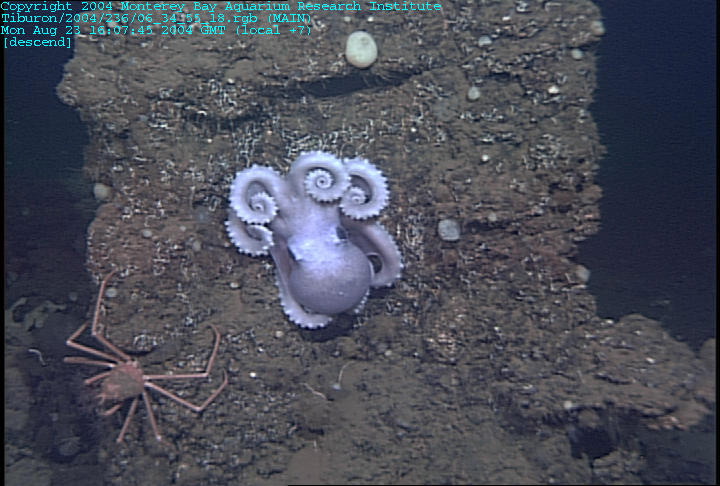
the Middle 150C Valley Field today with a full compliment of sampling devices in the ROV tool sled including major water samplers for collecting vent fluids, niskin bottles for bulk water sampling, push cores for recovering sediment samples down to 15cm, a biobox for bringing back rock or invertebrate samples, and temperature probes.At Middle Valley, we visited five sites with diffusive flows. We explored the Northern part of Middle Valley including the Heineken Hollow and East Hill. Because the diffuse flows are sparse on a large flat field of sediment and we have only a basic map of the seafloor, we used the sonar on the ROV to locate structures to investigate. In this area we passed over many inactive sites as indicated by extinct sulfide structures, lacking diffuse flow and often covered with dead clam shells. This picture shows old sulfide occupied by an octopus. In contrast, when we found an active mound it was covered with bacterial mats, live clams, and a simmering of diffusive flow was apparent.Middle Valley has a very different macrofauna than the Endeavor or Mothra seqments of the Juan de Fuca Ridge. Clams dominate the diffuse flows in Middle Valley. While in Main Endeavor field and Mothra black smokers are dominated by tubeworms, limpets
|
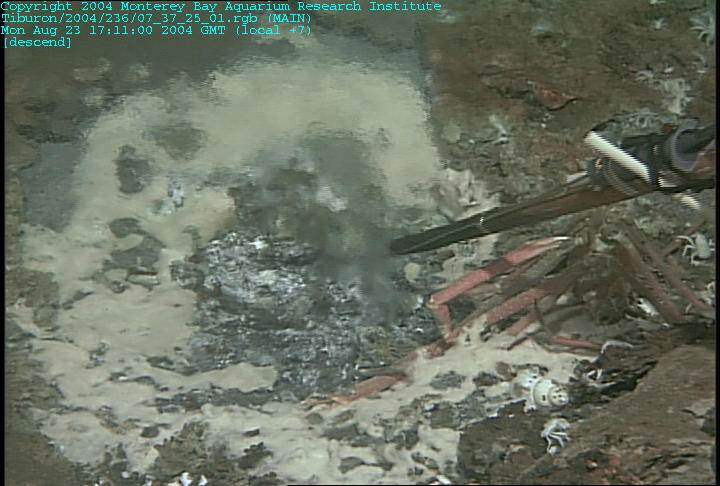
Dead Dog Mound, Central site and Inspired Mound taking additional water samples and using the temperature probe to get an idea of the temperatures of diffuse flows. For example, at Inspired Mound we found 150C fluids emanating from the chimney structure. Just an inch in the sediment below the chimney fluids were 98C, and water near the structure was between 1.6 to 6C. The temperature of the fluid drops rapidly as the cold seawater mixes with it
|
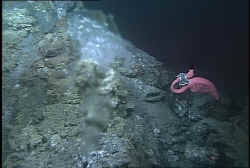
the Mothra and Main Endeavour vent fields. During these expeditions, we sampled high temperature flows from several different hydrothermal vent structures, collected fragments of hydrothermal vent chimneys, collected water from above diffuse vents, and completed transects to confirm high resolution maps made using acoustic instruments on prior cruises. Afterwards, we spent half a dive at a field called sasquatch. Like its namesake, this field can be difficult to find. However, the sasquatch vents were unable to hide from the keen eyes of our pilots, who found the site in no time (note: they did have some help from our colleagues who have produced exquisite maps of this area). In any case, this was also a wonderful dive because this field has an interesting structure. It sticks out of the seafloor like an artificial reef. Fish, like this skate, seem to be attracted to it (Do you think he''s looking for a meal?). It also gave us the opportunity to leave a marker at the site. This is a service to the scientific community because it allows us to find the same sites easily without having to waste precious time by flying around and trying to identify the vents by landmarks. We came up with a marker that is really quite distinct; one that I believe clearly designates the site. What do you think? I for one look forward to visiting Pinkie the Flamingo when we revisit this site in 2005 or 2006.
|
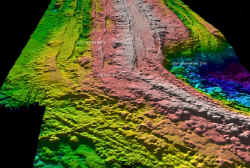
In fact, the presence of this assortment of evolved, water-rich rocksandesites and daciteshas been used to suggest that ophiolites could not possibly represent pieces of real mid-ocean ridges. Perhaps Dacite Hill will prove to be the missing link between ophiolites and mid-ocean ridges! The image to the left shows a perspective of the intersection of the southern Juan de Fuca spreading ridge to the north with its parallel crestal boundary ridges on either side of the spreading axis. On the bottom of the image is the ridge-transform intersection or RTI, where the ridge meets the westernmost Blanco Transform Zone. You can see that at this intersection the ridge wraps around the end of the transform in a series of hooked or curved ridges. Dacite Hill is one of the domes on these curved ridges, where the ridge is profoundly modified by the proximity to the cold transform zone. We look forward to the dive tomorrow to understand where our fragments of dacite originated. The Juan de Fuca Ridge crest and collect samples for study. One of the most exciting things about this cruise is the fact that we are going to be collecting samples very close to where the spreading ridge intersects the Blanco Fracture Zone; a junction known as the ridge-transform intersection or RTI. This is an exciting region to explore because previous expeditions have recovered dacites from the ocean floor in this area. Why is a dacite so exciting? Especially one found two miles under the surface of Pacific Ocean? Ocean floor dacites are exciting because they dont belong there. Its something that is unexpected and surprising and kind of reaches out and slaps you. It shouts out that something new and different is happening. Dacites are volcanic rocks that are high in silica (SiO2) low in magnesium (MgO) and are rich in volatiles such as water. Mid-ocean ridges (MOR) are places where extensive volcanism occurs. Imagine all the volcanoes on the continents erupting at the same time and you will still only have a fraction of the volcanism that occurs at MOR. Over 21 km3 of magma erupts each year at the MORs that circle the Earth. This is the system that creates new oceanic crust, that causes continents to rift and pull apart. This is a system that has been actively studied for many years and still provides new mysteries. The main geologic rock type that you expect to find at MORs, such as the Juan de Fuca, is a dark, fine-grained to glassy rock called basalt that erupts at temperatures around 1200C. These lavas are so common that they are called MORB, or mid-ocean ridge basalt. This is the first rock type that forms when you melt the mantle rock 10s of kms within the Earth. So, if MORB is so common then why is there dacite being found on the Juan de Fuca? One theory to explain this anomaly is that there was an isolated pot of magma that was allowed to cool slowly, removing more and more crystals, and it evolved from MORB to FeTi basalt to basaltic-andesite to ferroandesite and, finally, to dacite. This would require a closed system where nothing could disturb the pot while the evolution occurred. The other theory for the leap of common MORB to dacite is that there is something odd occurring at the RTI.The dacite was recovered near the RTI which scientists have hypothesized has a cold edge effect on magma evolution. Due to the transform offset of the ridge, the southern part of the Juan de Fuca, runs into a cold thick wall of oceanic lithosphere. This cold wall could cause the temperature of the magma chamber to drop further than expected and allow the magma to cool more and evolve further then it does at a normal ridge crest.The southernmost part of the Juan de Fuca Ridge where it intersects the Blanco Transform and the samples that it collects could help us determine what causes these highly evolved rocks to occur at the RTI.
|
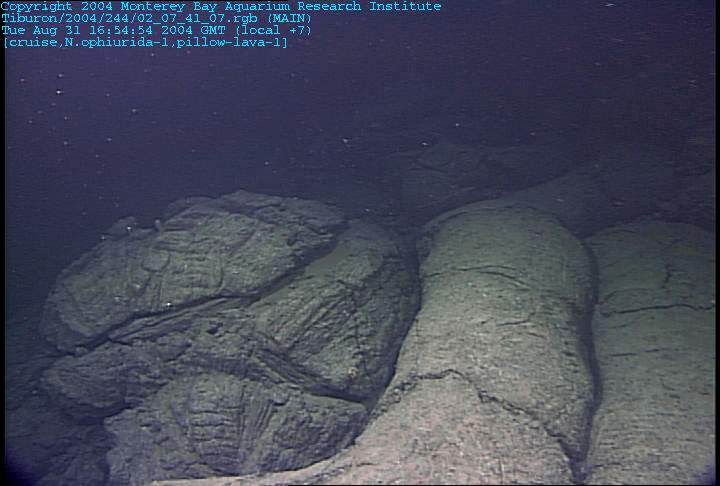
giant pillows, said Mike. Jim was just as certain that they would appear as thick sheets. So, we decided that we would look for and sample any lava that looked different, especially if it had lots of gas holes in it. Why the gas holes? Evolved rocks like andesites and dacites contain volatiles such as water and carbon dioxide dissolved in them prior to their eruption. When they do erupt the dissolved gas separates from the still viscous magma, leaving behind a hole where the gas bubble used to be. If the lava is viscous enough, the holes can get elongated or even flattened as the flow moves after the gas separates
|
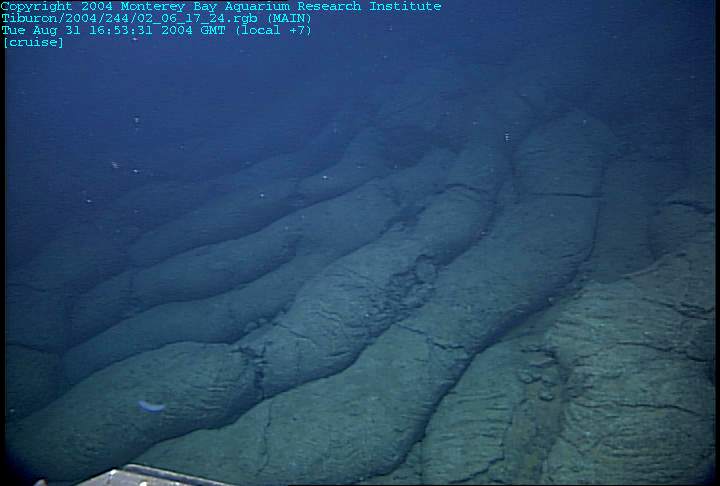
Dacite Hill so that we could climb up the side looking for anything different. This could prove to be a very long day. Near the bottom of the slope the rocks looked definitely normal with tectonized slices of broken pillows, which is in fact EXACTLY what you would expect to find in a transform zone. A large pillow or two was seen, but still nothing extraordinary. Then we began to see unusually fresh rocks with common morphologies, such as giant pillows and long lava tubes. These were topped by astonishingly perfect elongate pillow tubes
|
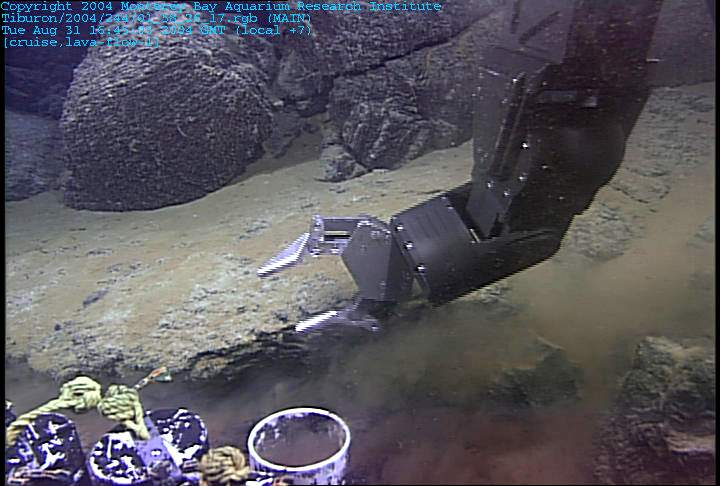
The summit of Dacite Hill was in fact a dacite dome with sheets and thick layers of gas-rich dacite
|
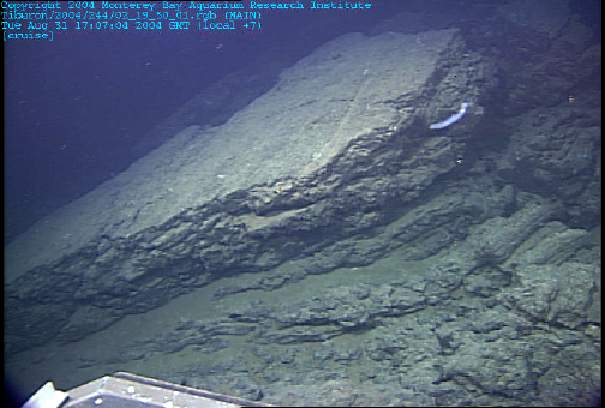
The peculiar structures were related to the viscous nature ofthe lava that do not flow easily and in some places could only push the surface rocks up into a dome-like structure. The day was completed by tracing the transition of the silicic rocks into the more normal structure of the southernmost spreading center.
|
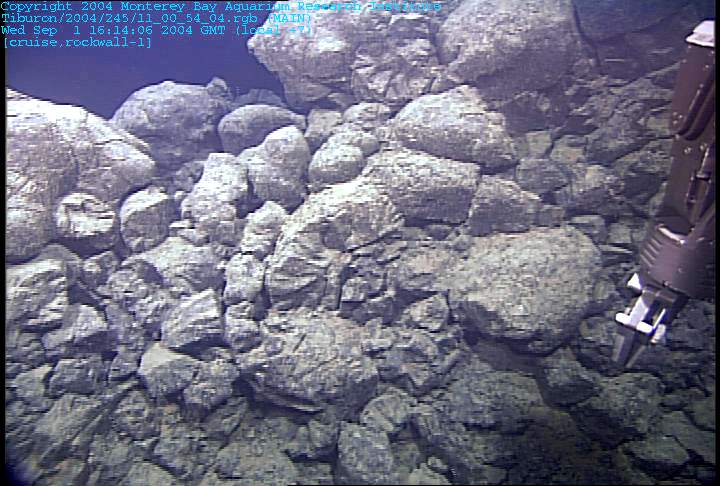
An investigation that looks at the geology to the east and west of the volcanically active axial valley. Model for south Cleft proposes that significant tectonic extension occurs within a couple of kilometers of the spreading axis, creating faults and deep open fissures that also provide conduits for magma from the cooler fringes of the subsurface magma chamber. By exploring the flanks we discovered that rocks erupted from the Cleft system actually include a broad range of compositions, representing magmas that had cooled to variable degrees. Dive T736 was begun within the axis just to get our bearings but almost immediately moved into the deformed zone characterized by thin slivers of sliced crust (see image to left). Some of these open fissures have pillowed lava flows that appear to have flowed over or into the fissure during its early stages of formation (see image to right). We noticed even as early as 2000 that almost all of the faults and fissures seemed to have piles of younger pillows along their length. Perhaps these pillows had been erupted from the fissures before they fully opened? The lavas within the axis of cleft are all flat sheets of lava sometimes twisted into folds and collapsed into pits. However the outer walls and flanks are dominated by big pillows, some of which drain and collapse like this one with crinoids along the edge. Fault
|
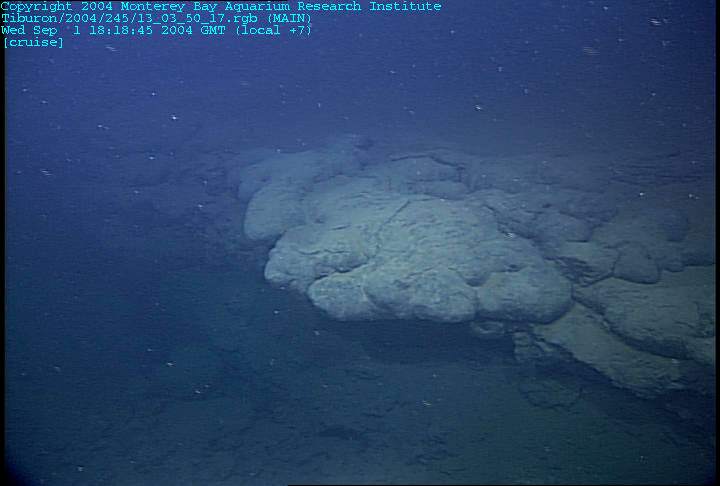
Fault. the same pattern of the flanks built of giant pillows. However we also found many large volcanic cones built right on the edges of the fissures. These little cones called hornitos (photographed in image to the right) are evidence of a local point source eruption of lava distinct from the eruptions within the axial valley. We saw several series of such hornitos before the flank became buried in sediment. However, halfway out the flank we found an extensive section of old sheet flows and collapse pits that were not covered by pillows. How could such flat flows still be so exposed three kilometers from axis? The skylights are like open cavities within a drained flow and should have been filled with sediment at least. Why arent these flows covered with pillows?
|
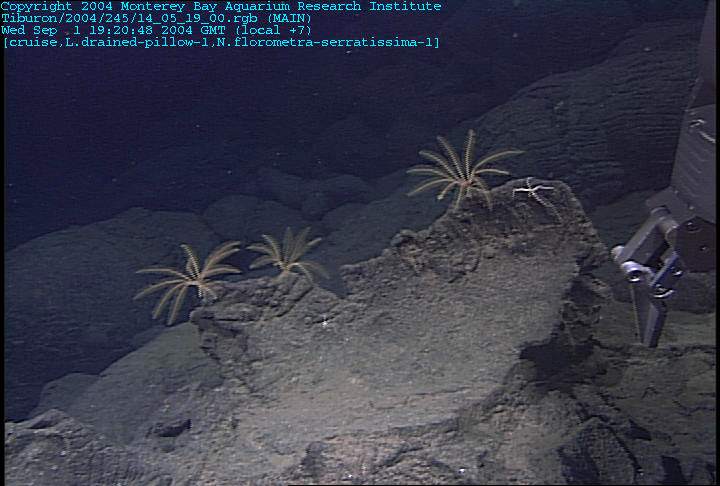
Fault. Crinoids.
|
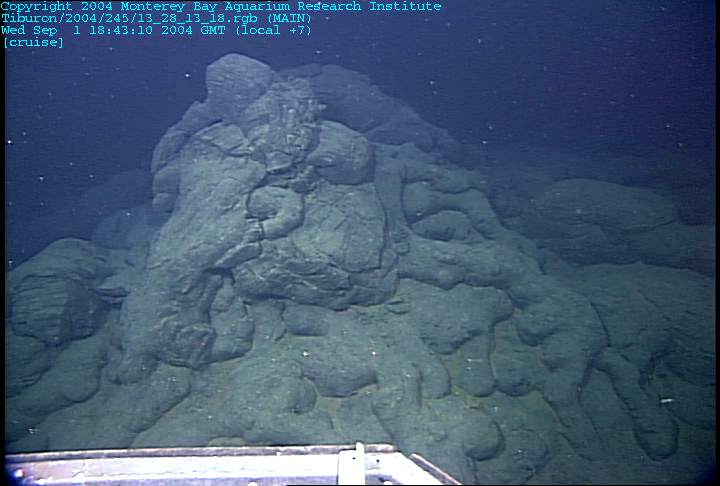
|
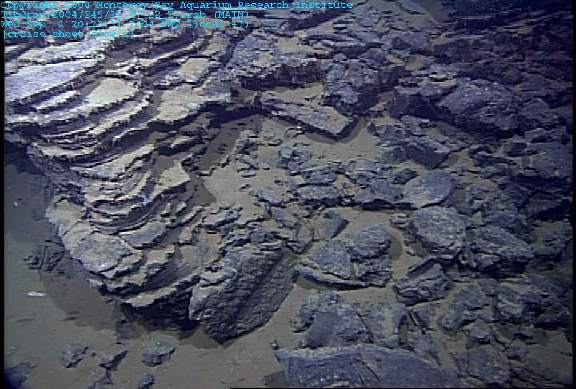
old sheet flows with a collapse pit to the right
|
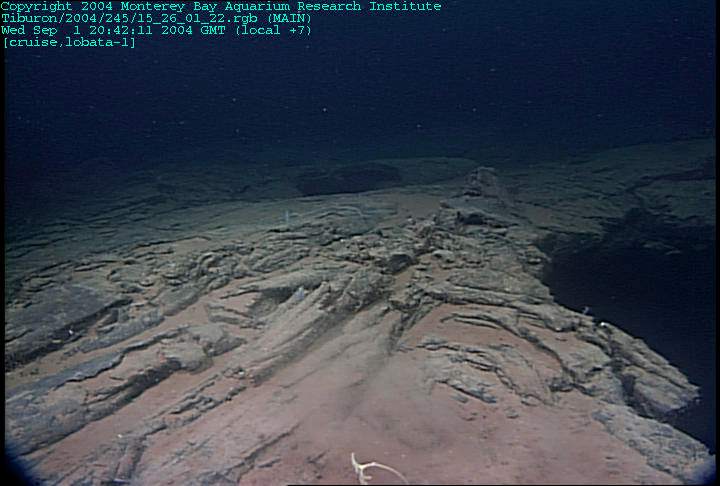
|
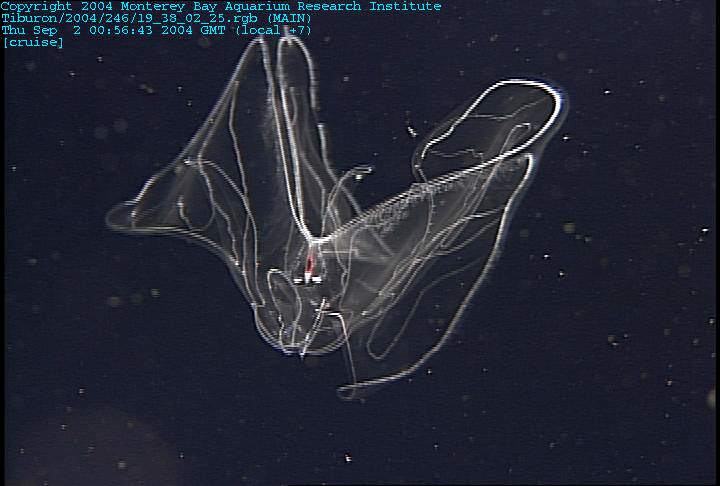
Even five and a half kilometers from the axis, there were still blocks of lava visible, although most of the seafloor was covered by sediment. As we drove along the extended stretches of sediment searching for these occasional outcrops, deep sea animals appeared to entertain us such as this beautiful comb jelly (Bathocyroe - a lobate ctenophore).
|
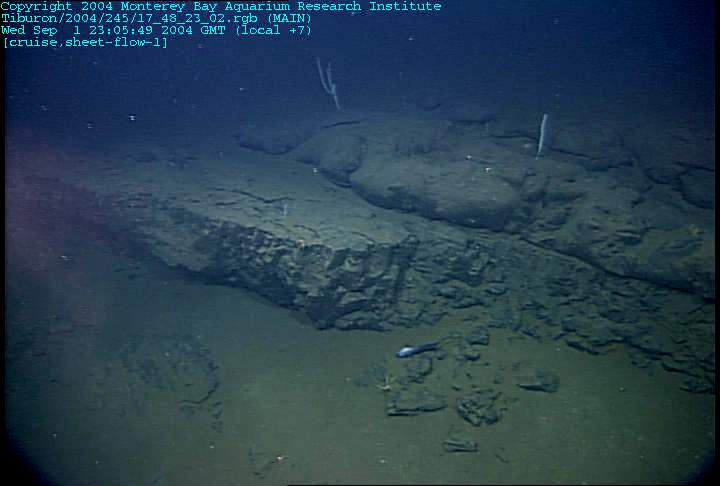
|
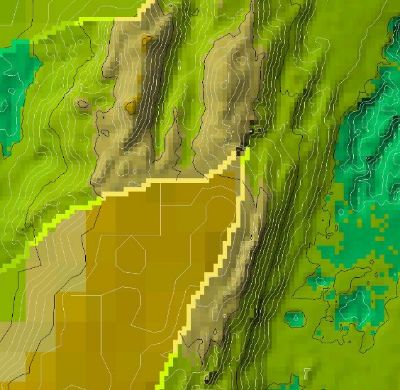
The whole Endeavour Segment has been mapped at the highest resolution possible with a ship, which means about 30-50m. This means that any seafloor feature smaller than 30-50m, like a rock outcrop or hydrothermal chimney, cannot be seen on the map. It would be like looking at a map of your neighborhood all the houses would be distinguishable, but the trees, cars, and people wouldnt show up. However, many parts of the axial valley at Endeavour have also been mapped. The normal ship-based bathymetric data is on the lower portion of the image and it accurately shows in a golden brown the axial ridge.
|
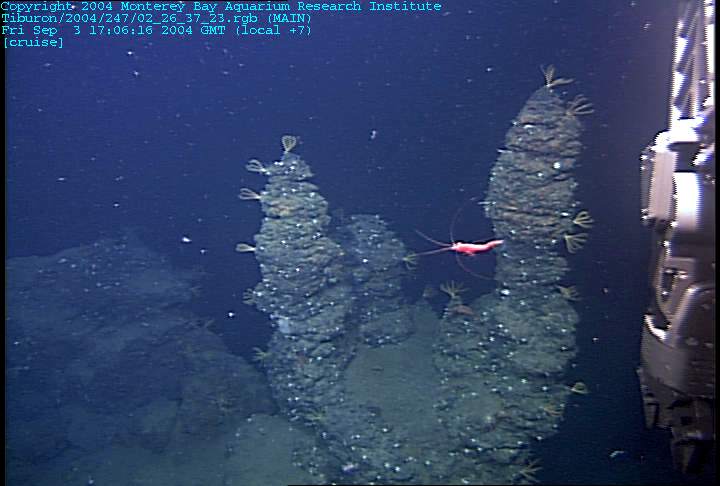
Everything was much bigger in scale than what we had seen at Cleft. The faults were more offset, the collapse pits were more extensive, and the hydrothermal deposits more abundant. We actually stumbled across a new low temperature vent and two inactive deposits in our one dive
|
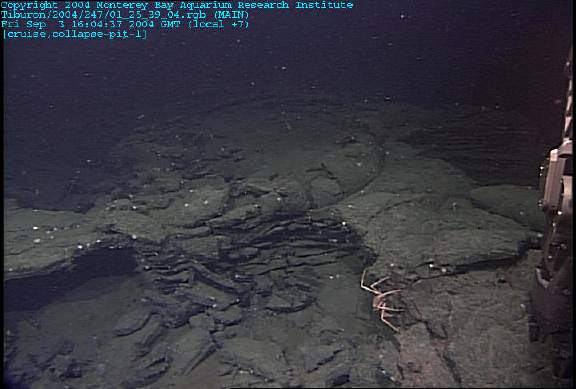
axis floor was filled with sheet flows and drained lava lakes. Such features indicate large volumes of lava being erupted to pond and then drain away. These drainage channels typically have tall pillars where steam or water was discharged. This is a picture of a very small pillar that shows the layers (see bottom right). Where the lava lake cools more completely you see thick massive layers of lava. This one seems to be surrounded by younger pillows. How do you think that it formed?
|
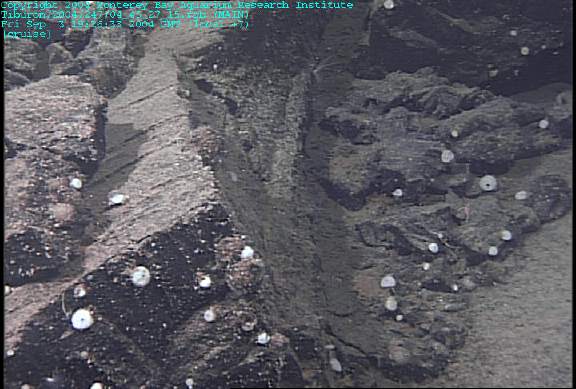
long transect across the entire axis including both flanks and the axial valley.
|
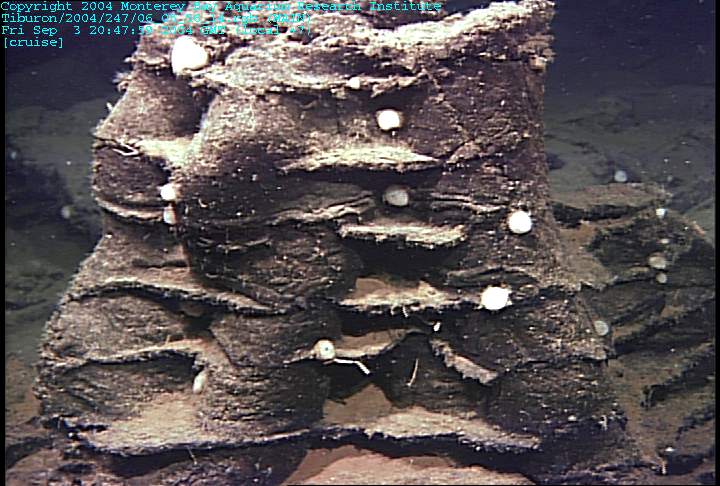
|
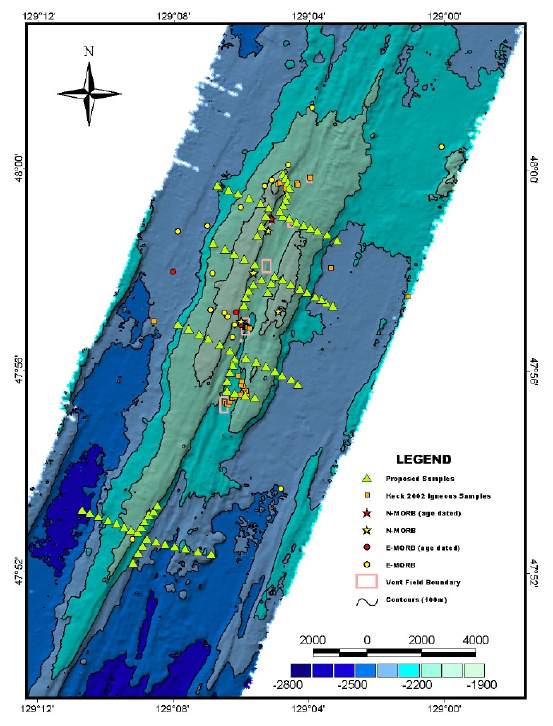
Endeavour Segment. On the map the two parallel ridges are a light green color with lighter green summits. The seafloor around the ridge is deeper and shows up as blues and grays on the map. Mid-ocean ridges are always shallower than the surrounding seafloor due to the thermal bulge of the upwelling mantle beneath. The mantle upflow is what delivers the small amount of melted rock that forms the ocean crust. Such mantle convection is one of the primary forces of our planet. The yellow triangles show all of the sites that we had hoped to sample, but we do not have enough time to accomplish all of those dive tracks. So together we must formulate a prioritized list. The plan that we finally decide upon is to spend the first dive crisscrossing the northern axial valley that has been the most neglected by previous studies. Remember that the axial valley is thought to contain the youngest erupted volcanic rocks and is thought by most geologists to be the ONLY location for mid-ocean ridge eruptions. Our next priority was to look at the range of relative ages and types of volcanism across the entire area. Today we essentially completed this transect, following the centermost line of yellow triangles shown on the map. Like true explorers we traveled a part of the seafloor that had never been seen before, recording our observations by digital imagery, slowly building up our model of how all of these volcanic events must have occurred to build such as edifice.
|
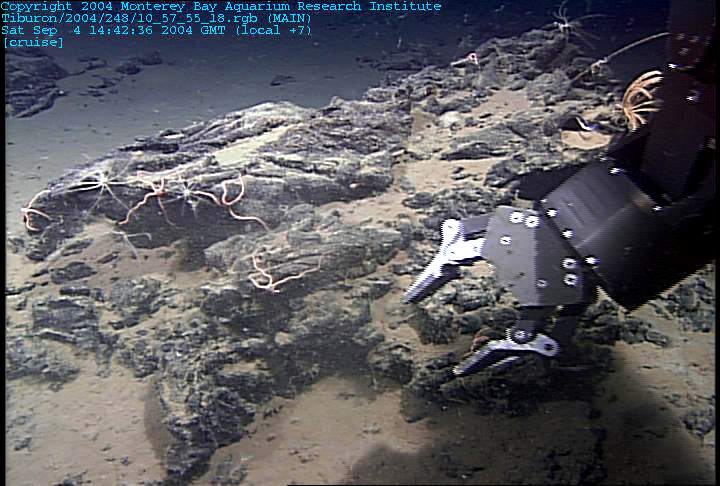
Folded sheet flows in most of the deeper areas
|
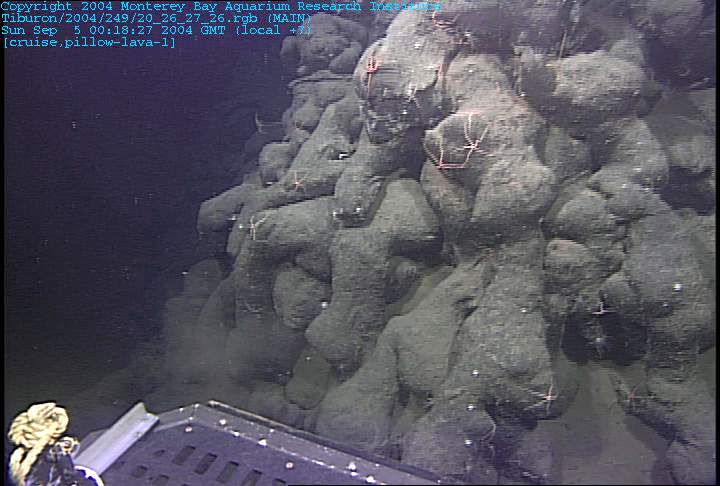
the steeper flanks built of piles of pillow tube
|
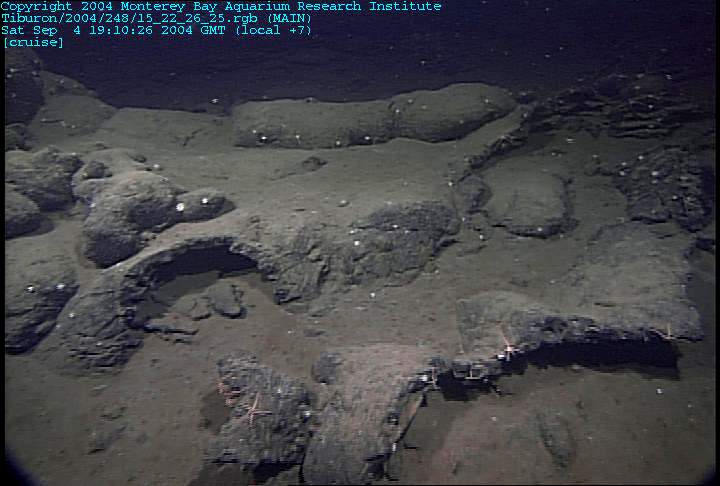
In between were areas of pillows drained of magma
|
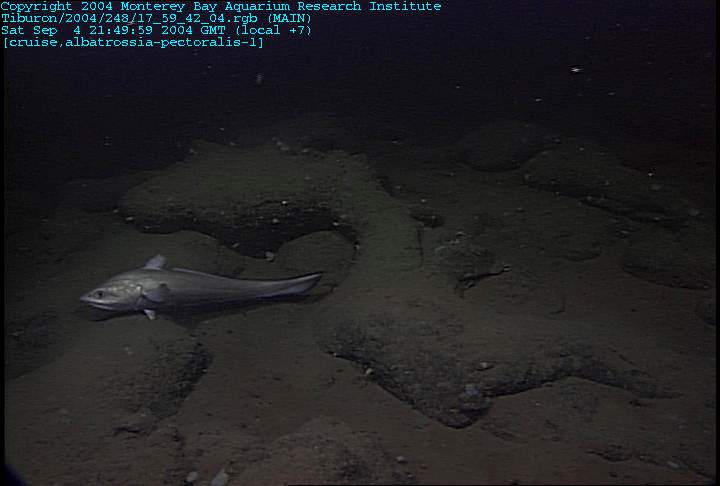
topped with flattened pillows
|
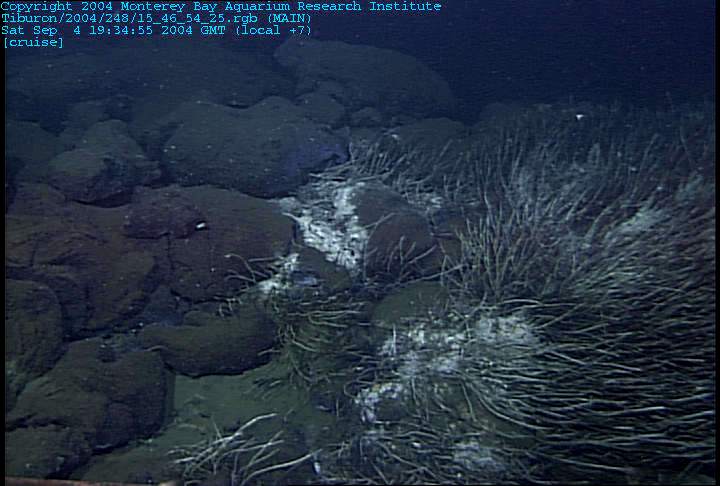
low temperature vent covered with graceful chemosynthetic tubeworms
|
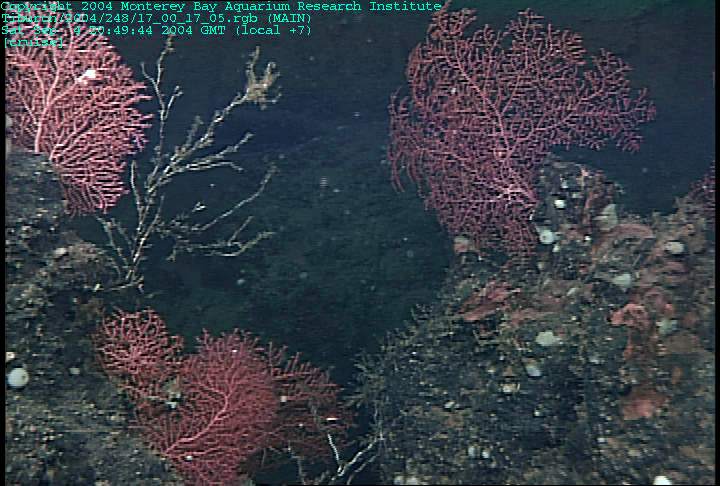
ld sulfide chimneys covered with animals including red seafans
|

Octopus
|
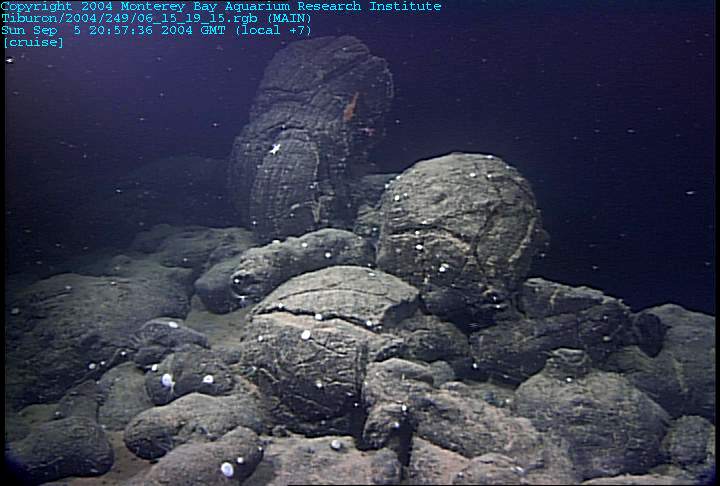
the High Rise vent field to west of the Main Field vent. This is the area that recent seismic imaging suggests has the most robust subsurface magma reservoir. Previous limited sampling also points to this area as having the greatest chemical variations that might be related to mixing and cooling within such a reservoir. The goal of the dive is to examine the geological evidence for magmatic injections into the ocean crust and to describe the morphologies of the lava flows for what clues could be provided. Previous dives in this area had provided glimpses into undescribed terrains of interconnected lava drainage channels, but no one knew their extent or continuity. The talus-covered east boundary wall of the axial boundary provided us with an easy to identify landmark, at least for that side. Interestingly, the lower portions of the fault had pile-ups of giant pillows that looked completely different from the axis floor (see photo to left). For the center of the axis we are dependent upon our navigation and the high-resolution bathymetry
|
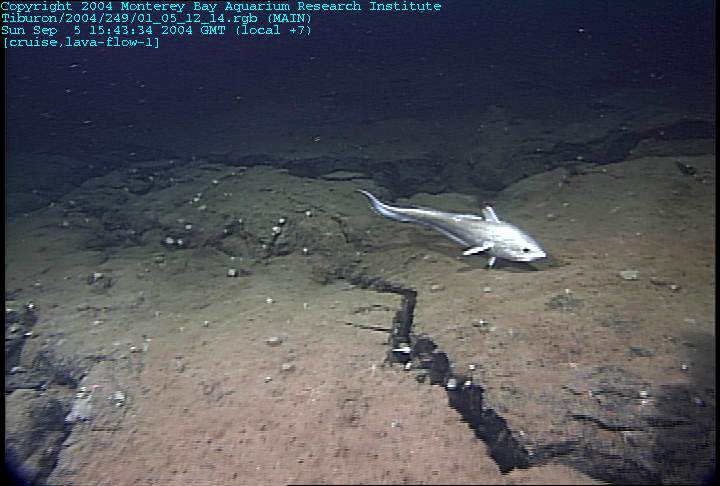
the magma maze. We found the deepest spots floored with expansive massive flows (see image to the right) that were cracked adjacent to the eastern fault
|
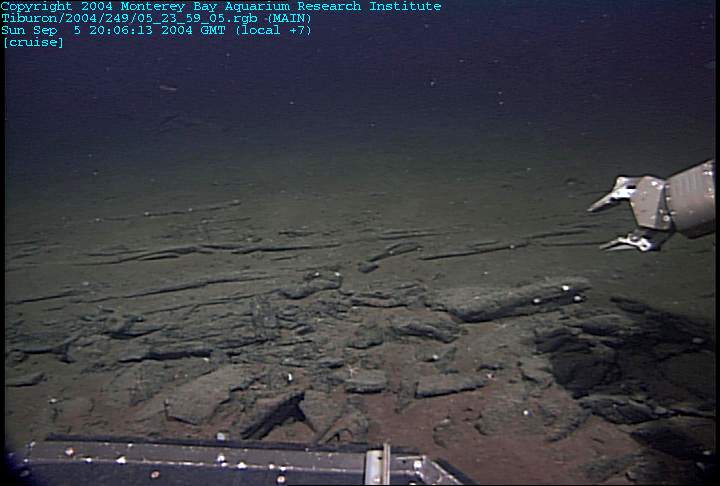
At the edges of the big sheets we entered the winding valleys of connected collapse pits bordered by drained lobate lava flows
|
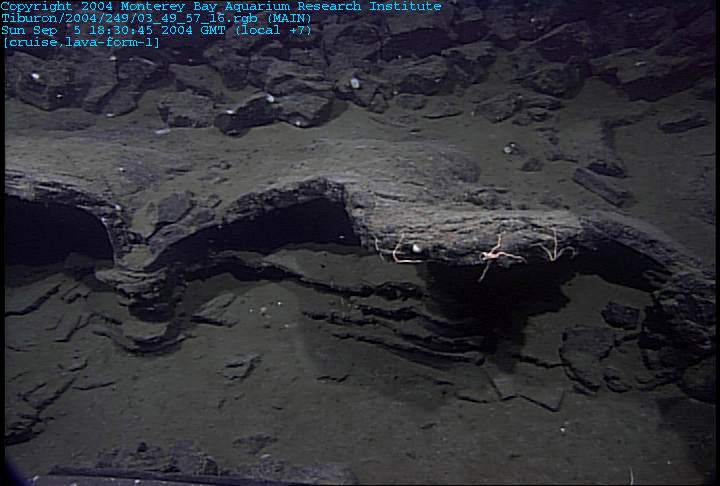
The drainage areas are characterized by abandoned towers of lava pillars sometimes attached to larger pieces of walls called buttresses
|
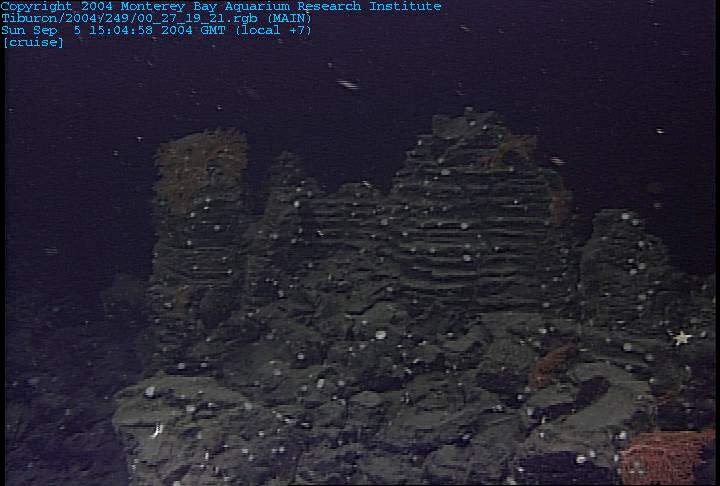
The drainage areas are characterized by abandoned towers of lava pillars sometimes attached to larger pieces of walls called buttresses
|
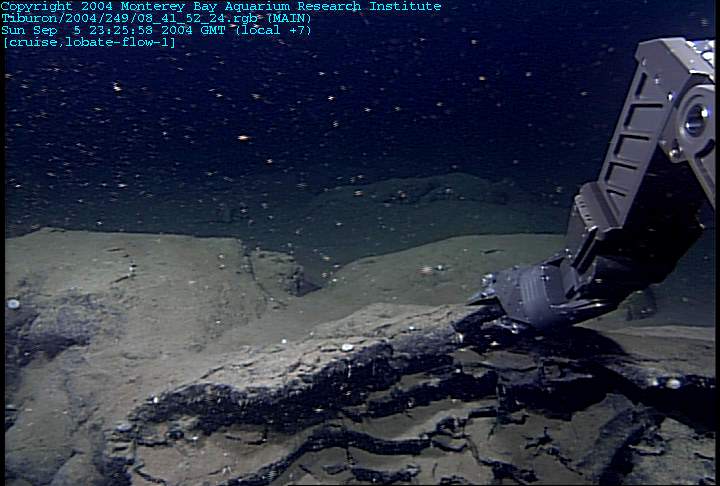
The glassy nature of these young basalts made them a challenge to sample using the manipulator
|
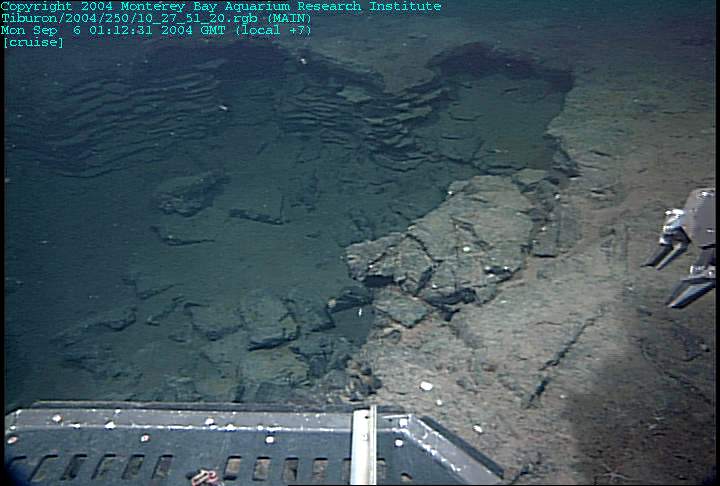
The cavernous nature of the collapse pits impressed everyone (see left image below). The most astounding recovery, however, came near the end of the day when a small slab recovered from this site revealed lava drips or stalactites on the bottom side when it was recovered. Submarine collapse pits and structures are common features found most prominently around the axes of all mid-ocean ridges. These collapse structures occur at a variety of scales from a few centimeters to 10s of meters. A good discussion of these features and their origin can be found in two papers by Mike Perfit, Jenny Engels and their colleagues published in 2003. The features we documented on the northern East Pacific Rise are very similar to what we have observed here on the Endeavor Ridge
|
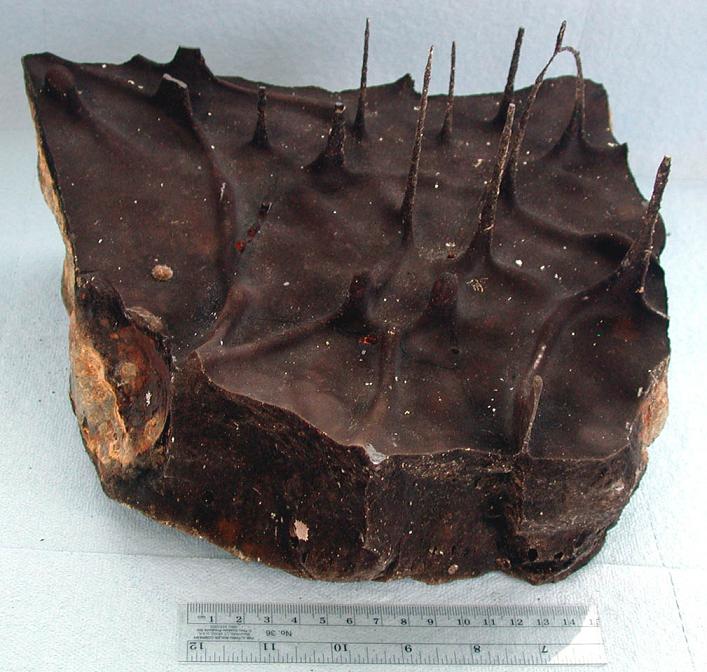
|
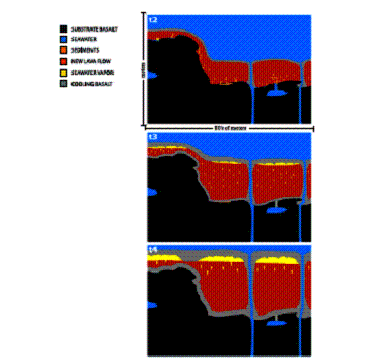
Engels Fig. 6b: This is a figure from the Engels, Edwards, Fornari, Perfit and Cann paper referred to in the Sept 5 update. A model for the process of lava lake collapse that we observed to be prevalent on the Endeavour ridge axis is described in this paper. You can get much more detailed information about this figure (and the next one) from the paper. You can think of these two figures as a timeline from when a lava lake starts to fill up at time 2 (T2) to when it drains and collapses at time 6 (T6). Each cartoon is a cross-section across the cooling lava lake. The colors represent specific things: the cold basalt rock basement is black, while the melted rock is red. The cold seawater is blue while the vaporized seawater is yellow. This figure shows how the seawater gets incorporated into the cooling lava flow at T2 and is vaporized to steam. The vapor bubbles (yellow) accumulate under the cooling lava roof rock (gray). The cooling roof rock (gray) forms drips or stalactites into the vapor cavities (yellow) as in our rock from Endeavour. Look at the large vapor cavities in T4 and compare these to some of the Endeavour pictures
|
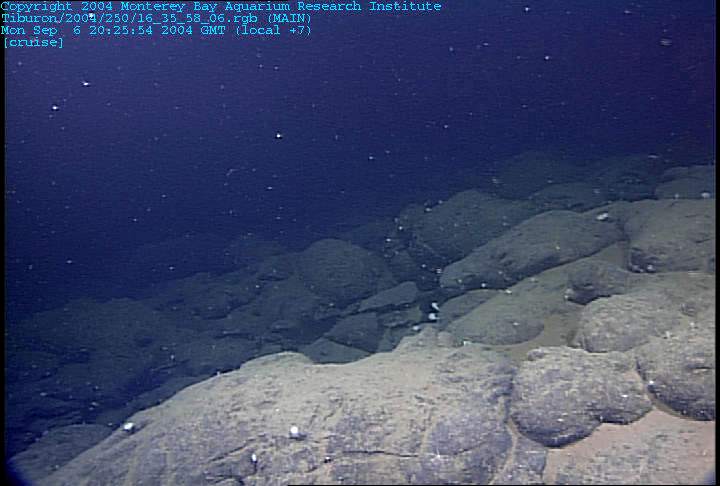
the Endeavour axial valley, we had some vision of the volcanic processes frozen in rock on the rift floor. We saw artifacts of great lava lakes rising and falling within the steep boundary walls. The walls themselves are more challenging to interpret because the pieces of ocean crust get very disrupted as they are sliced apart and pushed up into the high walls. Dive T738 provided us with a vocabulary and an inventory of potential types of volcanic constructs and dismembering faults. The last two dives for this expedition are focused on gathering field observations and rock samples to constrain the distribution of these volcanic rock types and their relationship to off-axis volcanism. Dive T740 was focused on the eastern flank of the ridge and Dive T741 will focus on the opposite western flank. We decided to examine the flanks adjacent to the central axis because this is thought to be the most volcanically robust section of the spreading segment. Dive T740 begins in the deep, sediment covered sheet flow east of the axis flanks. This type of flow is characteristic of the spreading axis and yet we find it way out here, completely barren of any cover of younger lavas. The rest of the Endeavour Ridge however becomes more explicable with each dive. The outward facing ridge flanks themselves are continuous pillow mounds covering very steep walls. We look at the direction of elongation of the pillow tubes to decide the downhill direction when the pillows were erupted (see image top left). Some of the pillow tubes actually flow nearly vertically downhill clinging to the steep fault face wall. The hornitos on the outer flanks are unique. There are lower pillows that have cracked open and poured out smooth thin flows that remind me of a roll of ribbon
|
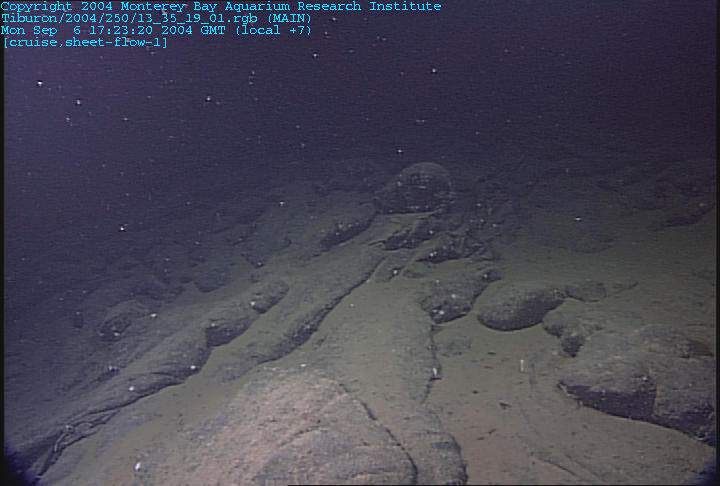
At the next level, pillows are oval and covered with glassy buds where the interior lava popped through the crust and leaked away. The last gasp of the vent is a single incredibly inflated pillow shape
|
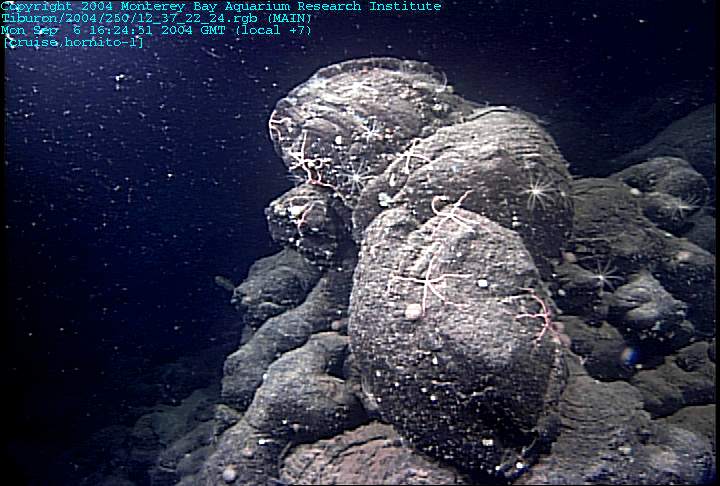
This succession likely has something to do with the temperature and rate of effusion of the lava, combined with the vent becoming filled with time. Clearly this orderly stack of volcanic structures must have erupted exactly in the spot that we find itoff-axis building up the outer flanks of the axis. On this dive we wander off our trackline for a few hundred meters parallel to the spreading axis to determine whether these volcanic features are continuous along the sides of the ridge axis. There are alignments of the giant pillows
|
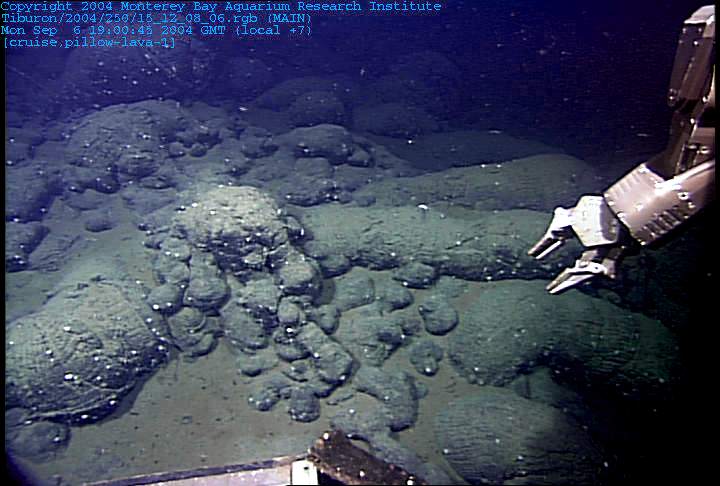
faulted slopes-This succession likely has something to do with the temperature and rate of effusion of the lava, combined with the vent becoming filled with time. Clearly this orderly stack of volcanic structures must have erupted exactly in the spot that we find itoff-axis building up the outer flanks of the axis. On this dive we wander off our trackline for a few hundred meters parallel to the spreading axis to determine whether these volcanic features are continuous along the sides of the ridge axis. There are alignments of the giant pillows
|
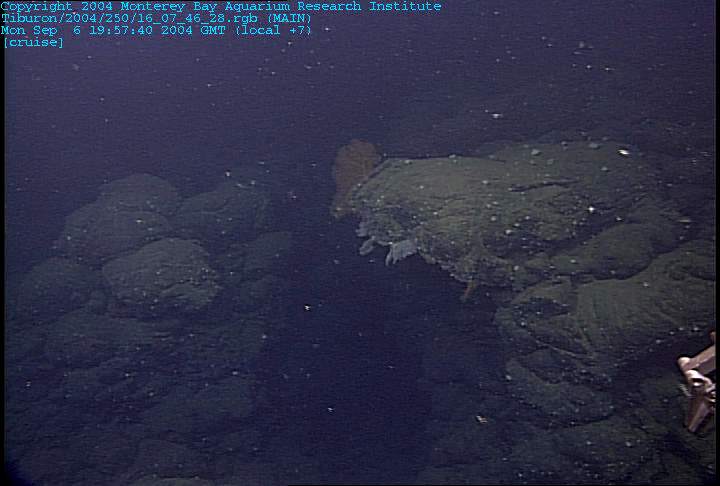
faulted slopes -pillows pouring into cracks
|
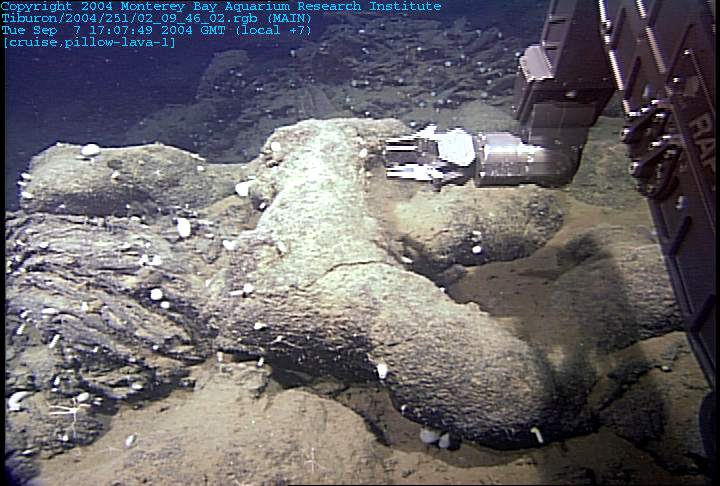
some underlying linkage between the faults and the volcanic activity. We complete our dive by driving over the top of the ridge and dropping into the axial valley floor. The inward facing wall was mostly great slopes of fault debris and avalanche chutes of pillow talus rolling downhill. BUT, every once in a while, we would get a peek at an outcrop of layered rocks, like the valley floor, topped by pillows, like the outward flanks
|
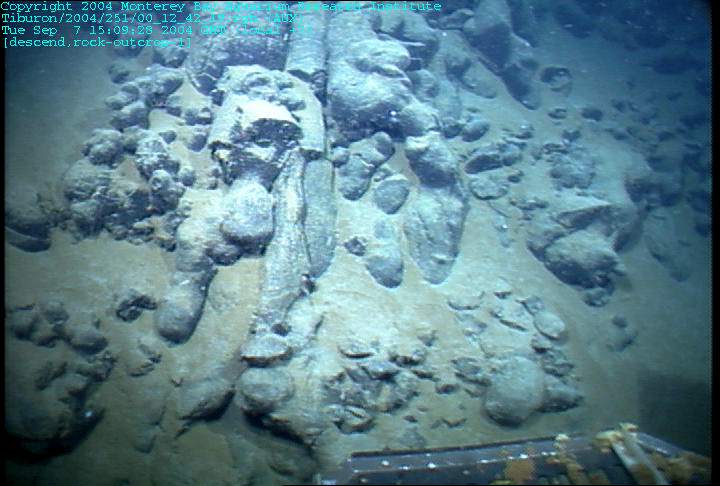
Dive T741 and the last dive for the Endeavour Segment, the Endeavour axial geology. We had thought that the western deep was completely covered wit h sediment, but our ROV pilots placed us right on top of a small mound of unbroken sheet flows. The bottom of the scarp includes both round pillow talus broken from overlying pillow tubes as well as in-place ribbon flows disgorged from the centers of large collapsed pillows. All of this is on top of the sediment showing to us that it had to be a pretty recent event unburied by the continual rain of sediment particles. We drove to the east straight up the wall and observed the same steep walls of pillowtubes hanging on the west flank that were so abundant on the east flank. The same variety of pillows was apparent with very fluid looking ribbon flows on the bottom, drained oblong pillows on top of the ribbons, and spectacularly elongate pillows clinging to the steep outward facing west flank wall. This pile of pillows has become diagnostic of the outer flank eruptions, which thicken the upper crust. As the ROV neared the summit of the western flank ridge we began to search for the sheet flows that are diagnostic of the fissure eruptions within the axial valley. We need more field evidence for the relationship between the pillow forms and these flat or folded lavas. The ridge top is cross-cut by fissures that are somewhat parallel to the orientation of axis of the ridge and suggest to us these fractures and faults are caused by the regional extension related to seafloor spreading. The outer flanks did not have such cracks or fissures why not? Could they have been completely covered by the pillows? If you look down into some of the open cracks you can just make out flat lavas down below on the wall capped by pillows. The low saddle between the pillowed highs revealed even more of the elusive sheet flows, now partially covered by the stringy pillows. The inward facing walls appear to be made of steps of the sheet flows from the inner flow neatly sliced and pushed up as if on an up escalator. These slices must be patiently waiting to get their cap of pillow lavas before they become part of the ridge flank.
|
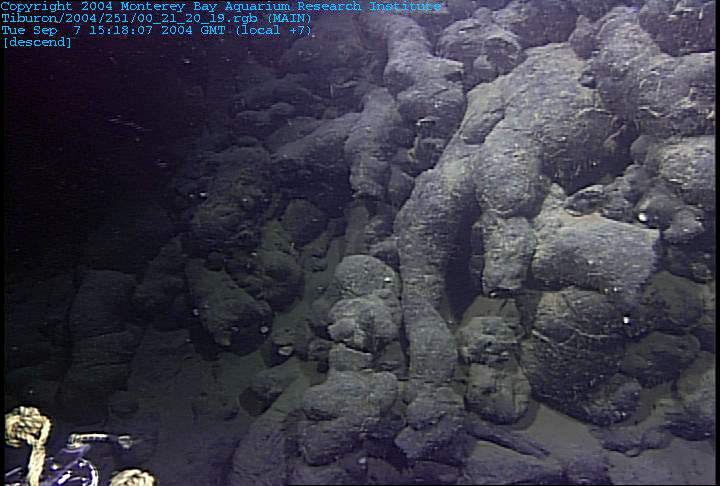
|
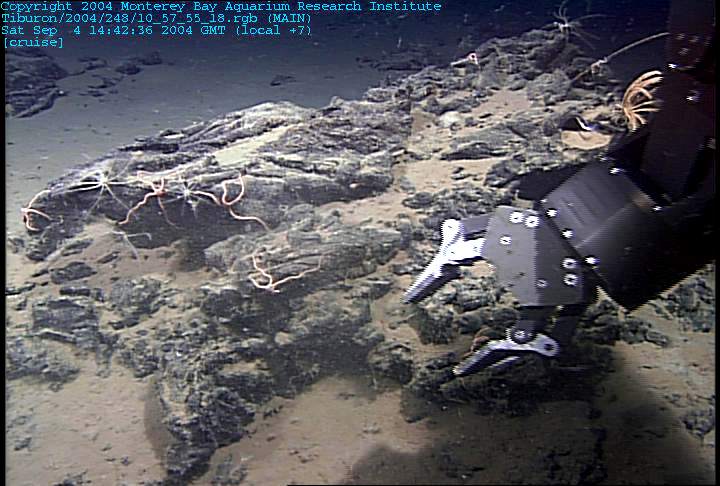
Folds
|
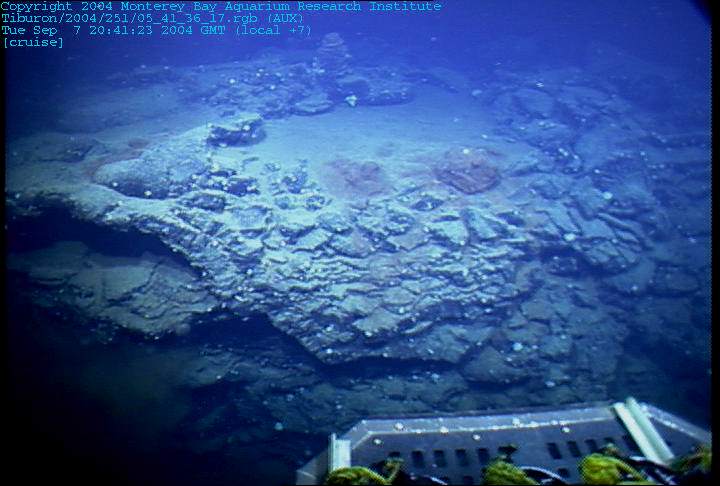
|
From MBARI -http://www.mbari.org/expeditions/WestCoast/logpages/August10.htm
Debra Stakes writes: After the disappointment of the previous dive, we decided to expand our search for potential seep sites to include two targeted dives in a single work day. Dives 473 and 474 were placed on the northern and southern sides of the Nootka Fracture Zone at its intersection with the Cascadia subduction zone. Each site would examine prospective sites of dewatering and or degassing of sediments as they are accreted to the subduction zone. We supposed that the transform intersection, by cleaving the accretionary slices, would induce zones of enhanced fluid flow. Dive 473 was placed along the northern wall of the Nootka "plunge pools" where the interior structure of the accreted slices would be exposed. The wall of the Nootka Fracture Zone proved to be many meters of tilted and somewhat deformed turbiditic sediments (Fig 1). Such sediments are characteristic of continental margins that serve as catchment basins for the eroding adjacent land. The movement into the subduction zone complex tilts the originally flat-laying sediments. Almost from the beginning of the dive, we observed dead clam shells mixed in with the eroding channels of siltstone and mudstone. But would we find the source of the shells today?
Near the summit of our vertical section, we observed an increasing abundance of cracks and fractures in the sedimentary rocks. Soon we were rewarded for our efforts with evidence of ongoing fluid flow in the form of groups of chemosynthetic tubeworms growing from the cracks (Fig. 2). At the top of the section, we finally discover groups of living clams nestled within one of the sedimentary layers. Both the sediments and the distribution of clams reminded me of the seeps in Monterey Bay (Fig 3). We mark the location with an acoustic beacon and head for the second area (Fig. 4)
Dive 474 covered much of the same sedimentary section as that observed on Dive 473. This dive transect covered the west-facing wall of the frontal accretionary wedge where the morphology suggested an extensive mass wasting event. Such events can expose deep stratigraphic sections with methane-rich pore fluids and permit these to vent laterally into the seafloor. We almost immediately found bunches of tubeworms attached to the broken sedimentary rocks (Fig. 5). Near the summit we found good evidence of methane seepage and oxidation - the cracks were filled with authigenic carbonate (Fig 6). We also found some local predators doing some seep faunal investigation on their own
Cleft Segment.
On the largest scale are features known as lava pond collapses that can be 10 m to greater than 100 m in diameter and kilometers long with very irregular shapes and cuspate edges. These are created by the filling and draining of lava lakes on the seafloor. They can be up to 25m deep but more typically less than 10m deep. These deep collapse channels are littered with pieces of the platy lavas that once formed the roof of the lava pond. Near the walls of the collapse tall spires of lava known at pillars, are common. In some places there is evidence of multiple levels of lava lakes; shallower ones will refill previous ones that have drained away. Smaller, semicircular holes commonly less than 10m in diameter and only a few meters deep are known as sky lights, similar to those observed over lava tubes on active volcanoes like those in Hawaii. On the seafloor these shallow collapse cavities join together in a network that may connect with the major collapse area in the lava pond. The smallest features, known as lava blister pits, generally represent the collapse of the top of individual lava lobes and are not interconnected.
Along with the observations that are made from manned submersibles and ROVs like Tiburon, we recover samples of lava from the various collapse features. The upper surface of submarine flows nearly always have a thick crust of black glass formed when the 1200C lava cools in seconds after coming in contact with near freezing 2C seawater. However, the undersides of these glassy crusts of lobate flows and sheet flows commonly have some very odd structures and textures that provide more evidence regarding the origin of the collapse. These features include lava drips, thin rivulets, flanges and bubble walls (see photos). All of these delicate forms require that the flows had to have open space beneath them in order for the lava to drip, and that the temperatures in this void space had to be high enough to allow the lava to remain molten, or at least plastic. This requires temperatures in excess of about 1070.
The question remains as to how and why these various collapse features form. The model that Perfit and his colleagues developed has to do with the interaction of extremely hot lava with seawater when there is an eruption. When lava pours out over the rough seafloor it covers spaces, cracks and fissures that are filled with seawater. When this trapped seawater is heated to temperatures over 400C, even at the great pressures on the seafloor, it forms a vapor (steam) and a liquid brine. If heated to 900C it becomes a salty steam that is so much less dense than the overlying lava that it bubbles up through the molten rock. However, because a glassy crust was immediately formed on the surface of the flow, the steam is trapped a few centimeters below the surface. Because seawater increases its volume by 2000% the rising bubbles can accumulate and create fairly large cavities. It is in these expanding hot cavities that the drips and bubble walls form (see Engels_Fig6b). Calculations suggest that these drip-filled cavities could form in minutes to hours -at about the same rate that flows are believed to thicken. The final key to the puzzle is that when the steam (and lava) finally cool, it condenses and contracts causing a decrease in pressure inside the cavity and the overlying pressure of 2500 meters of seawater to crush the thin crust of the now solid lava flow. In the deep collapse areas, much of the lava may drain away down the ridge or back into eruptive fissures, so when the roof collapses quite deep and extensive troughs remain (see Engels_Fig6c). Where the lava has flowed outward on to the surrounding seafloor as flattened lobates, the collapse is focused at the weakest central part of the roof. Chemical and experimental studies back on shore continue to try to solve this holey problem.
Here are some references if you would like to read more about this topic:
Chadwick, W. W., Quantitative constraints on the growth of submarine lava pillars from an instrument that was caught in a lava flow, J. Geophys. Res., 4, doi:10.1029/ 2003GC002422.
Chadwick, W. W., T. K. P. Gregg, and R. W. Embley, Submarine lineated sheet flows: A unique lava morphology formed on subsiding lava ponds, Bull. Volcanol., 61, 194206, 1999.
Clague, D. A., A. S. Davis, J. L. Bischoff, J. E. Dixon, and R. Geyer, Lava bubble-wall fragments formed by submarine hydrovolcanic explosions on Loihi Seamount and Kilauea Volcano, Bulletin of Volcanology, 61, 437449, 2000.
Engels, J.L., M.H. Edwards, D.J. Fornari, M.R. Perfit, J.R Cann, A new model for submarine volcanic formation, Geochemistry, Geophysics, Geosystems, 4, doi: 10,1029/2002GC000483.
Fornari, D. J., R. M. Haymon, M. R. Perfit, T. K. P. Gregg, and M. H. Edwards, Axial summit trough of the East Pacific Rise 9 10 N: Geological characteristics and evolution of the axial zone on fast spreading mid-ocean ridges, ,J. Geophys. Res., 103(B5), 98279855, 1998a.>
Perfit, M. R., J. R. Cann, D. J. Fornari, J. L. Engels, D. K. Smith, W. I. Ridley, and M. H. Edwards, Seawater-lava interaction during submarine eruptions at mid-ocean ridges, Nature, 426, 2003.
http://plate-tectonic.narod.ru/gordaphotoalbum.html |